2015 Young Investigator: Gary J Patti
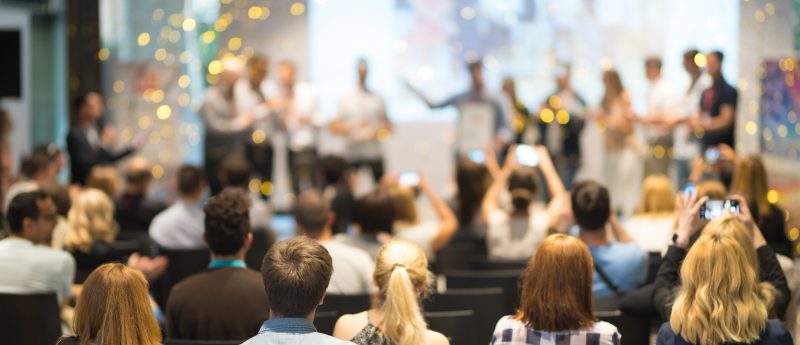
Nominee:
Nominated By:
Supporting Comments:
What made you choose a career in bioanalysis?
As an undergraduate, I remember taking biochemistry and learning how many ATP are produced from glucose. Our textbook said 38 ATP, but the professor said other sources said 36 or 32. Our entire class was based upon this number. I was shocked that such a seemingly simple (and important) bioanalytical measurement could not be made more quantitatively. I had just learned about NMR and MS. This seemed like a fantastic opportunity to me. Of course, what I didn’t recognize is how challenging it is to measure metabolites. Indeed, ever since I have been learning/developing technologies to do this (i.e., metabolomics).
Describe the main highlights of your bioanalytical research, and its importance to the bioanalytical community.
When we take an aliquot of standard human blood, extract its metabolites, and measure them with mass spectrometry, we find that the masses of over half of the compounds we detect cannot be found in any metabolite database or biochemistry textbook. A major effort of my research is developing bioanalytical technologies to identify these unknown compounds. Laboratories around the world have dense metabolic charts hanging on their walls displaying pathways that were mostly discovered in the 1950’s or before. We believe that these charts are incomplete and it is our goal to update them by discovering new metabolites and new pathways. Toward this end, we have tailored our metabolomic technologies for stable isotopes. In brief, we have developed a platform where we can track how labeled metabolite precursors are transformed unbiasedly. This has allowed us to answer some of the most fundamental biochemistry questions such as what are the complete fates of glucose in a cell. Additionally, by applying this platform, we have been able to not only identify unknown compounds, but also see how they are connected to other metabolites within our pathway maps
Describe the most difficult challenge you have encountered in the laboratory and how you overcame it.
When metabolites are extracted from biological samples and measured with MS there are thousands of masses detected that do not match any compound in the databases. Upon simple inspection, it seems like there is an overwhelmingly large number of “unknown” compounds. A good bioanalytical scientist, however, quickly realizes that many of the compounds detected are not real. That is, many compounds correspond to artifacts from sample contamination, instrument noise, MS fragmentation, non-biological transformations, etc. Given that the number of metabolites is unknown, how could we decipher which peaks were real and which were artifacts? It can take months to years to structurally characterize unknowns. Therefore, picking an artifact to go after could waste enormous time and resources. Yet, discarding data from compounds before they are identified seemed like an impractical objective.
Here again, we turned to stable isotopes. By mixing samples that had been grown in either 12C or 13C carbon, we determined that all compounds of biological origin have unique isotopic patterns in their mass spectra. This enabled us to “credential” real peaks for identification. While there are still many unknowns, this approach has enabled us to reduce the number of “unknowns” from thousands to hundreds.
Where do you see your career in bioanalysis taking you?
Thus far, my laboratory has largely focused on developing the analytical tools to track labeled metabolites unbiasedly. Now, we can dedicate our attention to applying this platform. Our goals are not only to identify new pathways, but also understand how they are regulated. I believe that biochemical regulation is an understudied area in which my laboratory can have a major impact by using metabolomic technologies.
In the traditional biochemistry textbooks, metabolic regulation is taught in what I call an ‘ATP-centric perspective’. It is presented with the assumption that a cell wants to maximize energy production. It is easy to find examples, however, in which this logic is incorrect. For instance, a rapidly dividing cell does not maximize ATP production. My goal is to understand regulation for these types of cells.
By using metabolomics, I would like to re-evaluate why cells make the metabolic decisions they do. While the fathers of biochemistry relied heavily on fresh muscle samples, we now have the capabilities to investigate metabolism using a myriad of exciting sample types and sophisticated label-tracking technologies. I believe that this will lead to the discovery of new metabolites, pathways, and regulatory phenomenon that will reform our understanding of basic biochemistry.
How do you envisage the field of bioanalysis evolving in the future?
With the emergence of cutting-edge bioanalytical technologies, we can now generate an enormous amount of data in a very rapid period of time. I believe that this ability to quickly generate big data sets is leading us to reconsider the fundamental scientific method. The scientific method says that you start with a hypothesis that can be tested with an experiment. The emergence of the omic sciences, however, have provided a platform for doing ‘hypothesis-generating’ experiments in lieu of these ‘hypothesis-driven’ experiments. Now, in many instances, it is more productive to generate data and then mine it to construct a hypothesis. Yet, at this time, hypothesis-generating ‘omic experiments are still not widely accepted as being scientifically rigorous and it can be particularly difficult to obtain funding for such laboratories. I believe that the future of bioanalysis is shaking this negative connotation of doing ‘fishing experiments’. I believe that as metabolomics matures and we can eventually integrate it with the other omic sciences, the power of big data will be undeniably compelling for doing untargeted analyses and will evolve to be a widely accepted version of the scientific method.
Please list up to five of your publications in the field of bioanalysis:
1. Patti GJ, Yanes O, Siuzdak G. Metabolomics: The Apogee of the Omic Triology. Nat. Rev. Mol. Cell Biol. 13(4), 263—269 (2012).
2. Mahieu NG, Huang X, Chen YJ, Patti GJ. Credentialing Features: A Platform to Benchmark and Optimize Untargeted Metabolomic Methods. Anal. Chem. 86(19), 9583—9589 (2014).
3. Patti GJ, Yanes O, Shriver LP, Courade JP, Manchester M, Siuzdak G. Metabolomics Implicates Altered Sphingolipids in Chronic Pain of Neuropathic Origin. Nat. Chem. Biol. 8(3), 232—234 (2012).
4. Chen YJ, Huang X, Mahieu NG, Cho K, Schaefer J, Patti GJ. Differential Incorporation of Glucose into Biomass during Warburg Metabolism. Biochemistry 53(29), 4755—4757 (2014)
5. Goedell L and Patti GJ. Maximizing the Value of Metabolomic Data. Bioanalysis 4(18), 2199—2201 (2012).
Please select one publication from above that best highlights your career to date in the field of bioanalysis and provide an explanation for your choice.
Patti GJ, Yanes O, Shriver LP, Courade JP, Manchester M, Siuzdak G. Metabolomics Implicates Altered Sphingolipids in Chronic Pain of Neuropathic Origin. Nature Chemical Biology 8(3), 232—234 (2012).
Here, we identified an ‘unknown’ metabolite called dimethylsphingosine (DMS) that was not previously known to occur naturally in biological systems. By using cutting-edge metabolomic technologies, we found that DMS concentration is increased in chronic pain. We have gone on to show that giving animals DMS is sufficient to induce pain and, more importantly, that blocking production of DMS can reduce pain. More recently, my laboratory has also shown that DMS is present in human tissue and increased in pain. We are now exploring DMS synthesis as a potential new target for pain.