Oligonucleotide pharmacokinetic/drug metabolism bioanalysis via hybridization ELISA
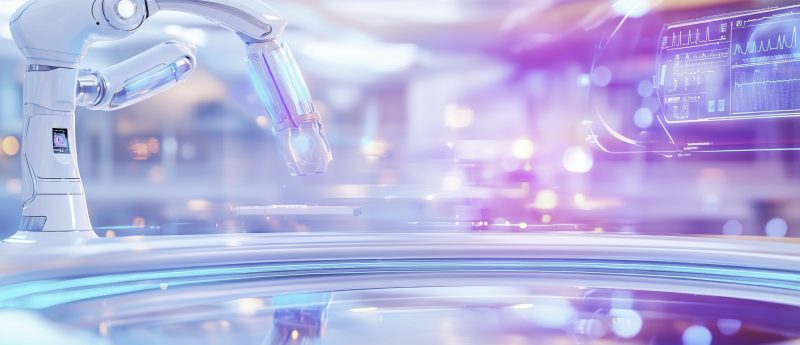
In this article, Eric Tewalt and James Gorman discuss updates and considerations for the utilization of hybridization ELISA methods, including associated benefits and challenges, for the bioanalysis of oligonucleotides.
About Eric Tewalt, Principal Scientist, Immunochemistry Department, PPD® Laboratories Bioanalytical Lab (Richmond, VA, USA).
Dr. Eric Tewalt serves as a Principal Scientist in the Immunochemistry Department at PPD Laboratories. He oversees a dedicated oligonucleotide team that conducts assay validation and bioanalysis of pharmacokinetic samples in support of sponsor clinical trials in a regulated environment. Dr. Tewalt has over five years of experience in the bioanalytical field and an additional nine years of experience in immunology, infectious disease and cancer research and has extensive knowledge in assay and experimental design. He received his Ph.D. in Immunology and Infectious Diseases from The Pennsylvania State University College of Medicine and conducted his postdoctoral research at The University of Virginia.
Introduction
The research and development of oligonucleotide analytes as pharmaceutical compounds for the treatment of a wide range of disease has been ongoing in academia and the pharmaceutical industry for well over 30 years. During the last 3 decades the field has expanded considerably to include antisense oligonucleotides (ASO), aptamers, small interfering RNAs (siRNA), microRNAs (miRNA), splice switching oligonucleotides (SSO), and more recently CRISPR/Cas9, among others [1]. Considerable advances in oligonucleotide chemical modifications also have increased target binding affinity and exonuclease resistance to increase in vivo half-life [2–8]. In addition, advances in the delivery of oligonucleotides to their target organs either through targeted administration (i.e., intrathecal, intranasal, intravitreal, etc.) [9–15] or via drug delivery mechanisms (i.e., GalNAc conjugation, lipid nanoparticles, etc.) [2,9,16 – 22], when combined with advances in chemical modifications [23], has increased potency thereby minimizing the dosing schedule as well as the amount of drug required to be efficacious.
There has been renewed interest in oligonucleotides given the recent approvals of Spinraza® (Ionis/Biogen) for the treatment of spinal muscular atrophy [24] and Eteplirsen (Sarepta) for the treatment of Duchenne muscular dystrophy [25]. The last 5 years has seen an increase in the number of pharmaceutical companies whose focus is on the development of oligonucleotide-based compounds. Further, the importance of oligonucleotide-based pharmaceuticals is evident as the majority of large pharmaceutical companies have oligonucleotides in their current pipelines either through partnerships or independent development. The allure is considerable given that oligonucleotides can be used to target a wide array of disease states including, but not limited to: cancer, viral infections and rare diseases(including genetic diseases), as well as numerous targets previously considered undruggable with traditional therapies.
Oligonucleotide bioanalysis platforms
Given the wide array of oligonucleotide-based compounds and the advances made in chemical modifications and drug delivery, the appropriate assay platform should be selected for pharmacokinetic (PK) profiling, drug metabolism and assessment of anatomical compound distribution. There are a number of assay formats that can be followed for PK analysis, among which chromatographic and enzyme-linked immunosorbent assays (ELISA) are typically favored. Liquid chromatography assays such as UPLC–UV, LC–FL, LC–MS/MS and LC–HRAM typically are highly selective, can distinguish between full-length oligonucleotides and their metabolites, and have a wide dynamic range, but in general require complex sample preparation and have a lower sample throughput.
An alternative to chromatography-based methods are hybridization ELISA methods. Briefly, the oligonucleotide analyte in the sample is hybridized to complementary capture and, if needed, detection probes. Special care must be taken when designing capture and detection probes to ensure appropriate affinity for the oligonucleotide analyte and to minimize potential self-annealing. The assay is completed by addition of a detection reagent and substrate. There are three hybridization ELISA formats that are typically utilized: 1) ligation hybridization ELISA; 2) nuclease-based hybridization ELISA; and 3) dual probe hybridization ELISA. The immunochemistry department in the PPD Laboratories bioanalytical lab (PPD BioA Richmond, VA, USA) has been conducting hybridization ELISA analysis since 2001 to support PK/DM sample analysis for non-clinical GLP, GLP-like and clinical PK/DM submissions. PPD BioA ICD has developed, transferred and validated methods utilizing all three hybridization ELISA formats.
Ligation hybridization ELISAs, which require an intact 3’ end on the oligonucleotide analyte, utilize a single template probe that binds the oligonucleotide analyte leaving an overhang at the 5’ end. A ligation probe, which is complementary to the 5’ overhang of the template probe, is then hybridized to the oligonucleotide analyte by T4 DNA Ligase prior to readout [26,27]. Nuclease-based hybridization ELISAs utilize a single labeled complementary cut probe that is biotinylated at one end and labeled with a detection tag at the other end to hybridize the oligonucleotide analyte. S1 nuclease is then added prior to readout to digest any single-stranded nucleic acids and increase selectivity for the parent compounds [28,29]. Dual probe hybridization ELISAs utilize a biotinylated capture probe that is complementary to a portion of the oligonucleotide analyte. After binding with the capture probe, a labeled detection probe that is complementary to the unbound portion of the oligonucleotide analyte is then added prior to readout [30].
Benefits of hybridization ELISA analysis
There are numerous benefits to utilizing hybridization ELISA analysis for PK/DM profiling. We have found, in collaboration with our sponsors, that hybridization ELISAs are appropriate for use across a wide range of oligonucleotide analytes (ASO, aptamers, siRNA, SSO, TLR agonist/antagonist, etc.), matrices (plasma, urine, tissue, etc.), and delivery mechanisms (lipid nanoparticles, GalNAc, polymers, etc.). Hybridization ELISA readouts also can be customized to suit specific bioanalytical needs and include, but are not limited to, absorbance/colorimetric, which most commonly utilizes streptavidin-HRP, and fluorescence intensity, which most commonly utilizes anti-Digoxigenin antibodies labeled with alkaline phosphatase. The advent of novel hybridization ELISA readouts and techniques are expected to expand as technological advances continue. Thus, hybridization ELISA is suitable for analysis of oligonucleotide analytes regardless of the nature of the compound, delivery mechanism or matrix.
In addition to global use of hybridization ELISAs for oligonucleotide analyte analysis, hybridization ELISA analysis provides the most sensitive platform for PK/DM analysis of oligonucleotide analytes. Sensitivity at the picogram/picomolar level can be achieved for hybridization ELISAs with appropriate accuracy and precision. Indeed, hybridization ELISAs are robust assays that exhibit exceptional accuracy and precision profiles (with %CV ≤ 20% and the accuracy within ± 20% of the theoretical value) and meet acceptance criteria set forth in the May 2001 FDA Guidance for Industry – Bioanalytical Method Validation [31]. The ability to produce even more sensitive hybridization ELISA formats will continue to be of the utmost importance given the recent advances in chemical modifications and delivery mechanisms. As discussed above, advances in drug delivery and chemical modifications to increase oligonucleotide analyte half-life have led to increased potency, resulting in the need for fewer doses and reduced amounts of drug required per dose. When combining the advances made in chemical modifications and targeted delivery, which can be synergistic [23], it becomes clear that reducing assay sensitivity will be a focus for hybridization ELISA formats moving forward. Along these lines, assays will need to be developed and optimized to reduce background noise to ensure appropriate response separation from blank matrix. This can be achieved through probe design and optimization of wash buffers, incubation temperatures and incubation duration or through technological advancement. Achieving a lower sensitivity could reduce the dynamic range of the assay by truncating the upper limit of quantitation (ULOQ). However, while the lower limit of quantitation (LLOQ) cannot be reduced, the ULOQ can be extended by demonstrating dilutional linearity that meets appropriate accuracy and precision standards. We have found, in collaboration with our sponsors, that dilutional linearity for hybridization ELISAs typically can be established upwards of 40,000 fold depending on the anticipated Cmax and can be further extended if needed during sample analysis. However, as the LLOQ is decreased due to advances in chemical modifications and targeted delivery, it also can be expected that the anticipated Cmax level will decrease as well causing a downward shift in the needed quantitation range of the assay. Thus, it is likely that the current ULOQs can be reduced, although they can be extended, if needed, through established dilutional linearity.
The use of hybridization ELISA also provides a number of benefits when conducting bioanalytical sample analysis. As opposed to chromatographic-based methods, minimal sample clean up or extraction is required. No sample extraction is required when using liquid-based matrices, such as plasma and urine. However, analysis of tissue samples does require homogenization and sample extraction, In general, hybridization ELISA analysis requires minimal sample volume typically in the 20–25 μL range. This allows for smaller patient blood draws and increases the ability to conduct any needed sample re-analysis (i.e., due to samples that do not quantitate within the established quantitation range) and incurred sample re-analysis. The minimal sample volume required also is beneficial when analyzing pre-clinical samples where sample volume is minimal (i.e., rodent studies) or from rare matrices, such as vitreous humor and cerebrospinal fluid. The sample volume required potentially can be further reduced through utilization of an appropriate minimum required dilution, established during assay development.
Further, hybridization ELISA offers high throughput sample analysis. This is due in part to simultaneous sample readouts being available as opposed to the linear injection process required for chromatographic platforms. In addition, hybridization ELISA analysis is usually conducted in a 96 well format with the ability to analyze approximately 30–35 samples per plate depending on layout. Additional advances to allow analysis to occur in the 384 well plate format would allow for further increases in sample throughput. When coupled with the minimal amount of time to conduct a hybridization ELISA (approximately 3–5 hours), thousands of samples can be analyzed over a period of 3–5 days. Sample throughput potentially can be increased with the use of automation, for which hybridization ELISAs can easily be adapted. Indeed, we have found that the use of automation provides a means to prepare calibrator and quality control preparations thereby reducing variability resulting in better accuracy, precision and method reproducibility. In addition, automation is heavily utilized to conduct standard sample dilutions saving time and manual effort. It should be noted, however, that appropriate process stability should be established if specific steps or incubations are automated and that automation should be customized for each specific hybridization ELISA method.
Challenges of hybridization ELISA analysis
While there are a multitude of benefits to using hybridization ELISA formats, there are also associated challenges. The majority of chromatographic-based methods are highly selective for the parent compound and can distinguish and measure metabolites. However, hybridization ELISA methods do not discriminate between quantitation of full-length oligonucleotides and metabolites. Thus, it is imperative to determine during assay development and validation if the hybridization ELISA method is selective for the parent compound or if metabolites potentially quantitate as well. It is recommended that a variety of shortened metabolites be analyzed during validation to assess which, if any, will quantitate. It is recognized that longer metabolites, such as N-1 and N-2, are likely to quantitate. However, these metabolites may maintain the ability to bind to the intracellular target and continue to have the desired therapeutic effect. Selectivity for the parent compound may be increased through appropriate probe design and the addition of S1 nuclease as described above. Metabolites also may interfere with the ability of the parent compound to quantitate appropriately. As with measuring metabolite quantitation, it is recommended that a cohort of metabolites of various sizes, especially longer metabolites, be assessed during assay development and validation for their ability to interfere with the quantitation of the full-length analyte. Finally, as opposed to chromatographic platforms, hybridization ELISA formats do not have the capability to distinguish and quantitate putative metabolites. The importance of the ability to quantify metabolites is evident as it is imperative to understand how quickly breakdown of the full-length oligonucleotide is occurring for dosing decisions, which metabolites are most prevalent and where the metabolite breakdown is occurring anatomically. There is the potential to develop hybridization ELISA methods to quantitate metabolites. However, with current methodology each metabolite would have to be quantitated via a separate method. When compared to the ability of chromatographic platforms to assess all metabolites in a single run, this is not financially feasible or timely to accomplish.
Considerations for the analysis of tissue samples
PPD BioA ICD has developed and qualified numerous hybridization ELISA methods for various tissue homogenates, including but not limited to the liver (targeted drug delivery) and kidney (analyte excretion). There are a variety of difficulties that are presented with the analysis of tissue samples. First and foremost is that as opposed to liquid-based matrices, sample extraction must occur for analysis of tissue samples regardless of the platform chosen for analysis. Typically this is accomplished through sample homogenization and some form of liquid extraction. However, the steps of sample homogenization must take into account the type of tissue sample. For example, soft tissues such as liver and kidney are easily homogenized using simple bead-based methods. However, organs that contain a high amount of connective tissue, such as the lungs, may need to be further processed or undergo multiple rounds of bead-based homogenization. The steps to fully homogenize tissue samples should be assessed during assay development prior to assay qualification, to ensure complete homogenization of study samples occur. Failure to fully homogenize samples could result in oligonucleotide analytes remaining trapped in tissues resulting in under recovery of sample quantitation. In addition, and from a regulatory standpoint, the qualification or validation of methods for analysis of tissue-based samples must be carefully designed. At PPD we recommend that tissue-based methods undergo method qualification using the same parameters as validation with modified stability. True sample stability, and in particular long-term stability, is extremely difficult to assess as drug product cannot easily be spiked into intact tissue much like it can in liquid matrices. Rather, the oligonucleotide can be added to the tissue sample prior to homogenization or to the sample homogenate following homogenization. We typically add the oligonucleotide analyte to the sample homogenate following homogenization so that, at the very least, sample stability following homogenization can be established. However, it is recognized that this does not cover the period of time from tissue harvesting through homogenization. Additional potential alternatives are to utilize study samples and assess the percent difference from the original freshly prepared result (similar to incurred sample reproducibility). Some considerations for this approach are whether the sample is harvested and the homogenate is split and compared, or if the tissue is minced and homogenized separately for each time point. If the tissue is minced and homogenized separately, then caution must prevail as it is recognized that oligonucleotides will concentrate differently depending on the anatomical location of the tissue sample (i.e., medulla versus cortex). The qualification and validation of tissue-based methods will continue to be a topic of interest, especially with regards to regulatory compliance and filing, as advances made in targeted oligonucleotide delivery continue.
Oligonucleotide hybridization ELISA experience at PPD BioA ICD
PPD BioA ICD has more than 15 years of oligonucleotide PK analysis experience and offers a wide array of services including assay development, validation and sample analysis to support oligonucleotide-based clinical trials. We offer multiple technology platforms to conduct PK sample analysis including, but not limited to: absorbance, fluorescence and electrochemiluminescent capabilities. As advances in the field of oligonucleotide-based therapeutics are made, PPD BioA ICD will continue to adapt and advance technologies and techniques in parallel to ensure the success of our collaborators.
2. Khvorova A, Watts JK. The chemical evolution of oligonucleotide therapies of clinical utility. Nat. Biotechnol. 35(3),238–248 (2017).
3. Prakash TP. An overview of sugar-modified oligonucleotides for antisense therapeutics. Chem. Biodivers. 8(9),1616–1641 (2011).
4. Watts JK. Locked nucleic acid: tighter is different. Chem. Commun. 49(50), 5618–5620 (2013).
5. Seth PP, Siwkowski A, Allerson CR et al. Short antisense oligonucleotides with novel 2’-4’ conformationaly restricted nucleoside analogues show improved potency without increased toxicity in animals. J. Med. Chem. 52(1), 10–13 (2009).
6. Cummins LL, Owens SR, Risen LM et al. Characterization of fully 2’-modified oligoribonucleotide hetero- and homoduplex hybridization and nuclease sensitivity. Nucleic Acids Res. 23(11),2019–2024 (1995).
7. Monia BP, Johnston JF, Sasmor H, Cummins LL. Nuclease resistance and antisense activity of modified oligonucleotides targeted to Ha-ras. J. Biol. Chem. 274(24), 14533–14540 (1994).
8. Choung S, Kim YJ, Kim S, Park HO, Choi YC. Chemical modification of siRNAs to improve serum stability without loss of efficacy. Biochem. Biophys. Res. Commun. 342(3), 919–927 (2006).
9. Geary RS, Norris D, Yu R, Bennett CF. Pharmacokinetics, biodistribution, and cell uptake of antisense oligonucleotides. Adv. Drug Deliv. Rev. 87, 46–51 (2015).
10. Miller TM, Pestronk A, David W et al. An antisense oligonucleotide against SOD1 delivered intrathecally for patients with SOD1 familial amyotrophic lateral sclerosis: a phase 1, randomized, first-in-man study. Lancet Neurol. 12(5), 435–442 (2013).
11. DeVincenzo J, Lambkin-Williams R et al. A randomized, double-blind, placebo-controlled study of an RNAi-based therapy directed against respiratory syncytial virus. Proc. Natl. Acad. Sci. U.S.A. 107(19),8800–8805 (2010),
12. Lee Du, Huang W, Rittenhouse KD, Jessen B. Retina expression and cross-species validation of gene silencing by PF-655, a small interfering RNA against RTP801 for the treatment of ocular disease. J. Ocul. Pharmacol. Ther. 28(3),222–230 (2012).
13. Solano EC, Kornbrust DJ, Beaudry A, Foy JW, Schneider DJ, Thompson JD. Toxicological and pharmacokinetic properties of QPI-1007, a chemically modified synthetic siRNA targeting caspase 2 mRNA, following intravitreal injection. Nucleic Acid Ther. 24(4),258–266 (2014).
14. Luu KT, Norris DA, Gunawan R, Henry S, Geary R, Wang Y. Population pharmacokinetics of nusinersen in the cerebral spinal fluid and plasma of pediatric patients with spinal muscular atrophy following intrathecal administrations. J. Clin. Pharmacol. doi:10.1002/jcph.884(2017).
15. Fattal E,Bochot A. Ocular delivery of nucleic acids: antisense oligonucleotides, aptamers, and siRNA. Adv. Drug Deliv. Rev. 58(11), 1203–1223 (2006).
16. Juliano RL. The delivery of therapeutic oligonucleotides. Nucleic Acids Res. 44(14), 6518–6548 (2016).
17. Lorenzer C, Dirin M, Winkler AM, Baumann V, Winkler J. Going beyond the liver: progress and challenges of targeted delivery of siRNA therapeutics. J. Control Release. 203, 1–15 (2015).
18. Evers MM, Toonen LJ, van Roon-Mom WM. Antisense oligonucleotides in therapy for neurodegenerative disorders. Molecules. 87, 90–103 (2015).
19. Nair JK, Willoughby JL, Chan A et al.Multivalent N-acetylgalactosamine-conjugated siRNA localizes in hepatocytes and elicits robust RNAi-mediated gene silencing. J. Am. Chem. Soc. 136(49),16985–16961 (2014).
20. Raney SG, Wilson KD, Sekirov L, Chikh G, de Jong SD, Cullis PR, Tam YK. The effect of circulation lifetime and drug-to-lipid ratio of intravenously administered lipid nanoparticles on the biodistribution and immunostimulatory activity of encapsulated CpG-ODN. J. Drug Target. 16(7), 564–577 (2008).
21. Davis ME, Zuckerman JE, Choi CH et al. . Evidence of RNAi in humans from systemically administered siRNA via targeted nanoparticles. Nature. 464(7291), 1067–1070 (2010).
22. Prakash TP, Graham MJ, Yu J. Targeted delivery of antisense oligonucleotides to hepatocytes using triantennary N-acetyl galactosamine improves potency 10-fold in mice. Nucleic Acids Res. 42(13), 8796–8807 (2014).
23. Prakash TP, Kinberger GA, Murray HM. Synergistic effect of phosphorothioate, 5’-vinylphosphonate and GalNAc modifications for enhancing activity of synthetic siRNA. Bioorg. Med. Chem. Lett.26(12), 2817–2820 (2016).
24. US Food and Drug Administration. FDA approves first drug for spinal muscular atrophy. www.fda.gov/newsevents/newsroom/pressannouncements/ucm534611.htm (Accessed 16 June2017)
25. US Food and Drug Administration. FDA grans accelerated approval to first drug for Duchenne muscular dystrophy. www.fda.gov/newsevents/newsroom/pressannouncements/ucm521263.htm (Accessed 16 June 2017)
26. Baker BF, Zhengrong Y, Leeds JM. Method for quantitating oligonucleotides. WO 2001034845 A1. (2001).
27. Yu RZ, Baker B, Chappell A, Geary RS, Cheung E, Levin AA. Development of an ultrasensitive noncompetitive hybridization-ligation enzyme-linked immunosorbent assay for the determination of phosphorothioate oligodeoxynucleotide in plasma. Anal. Biochem. 304(1), 19–25 (2002).
28. Yu Z, Baker B, Wu H. Nuclease-based method for detecting and quantitating oligonucleotides. WO 2002059137 A1. (2002).
29. Wei X, Dai G, Marcucci G, Liu Z, Hoyt D, Blum W, Chan KK. A specific picomolar hybridization-based ELISA assay for the determination of phosphorothioate oligonucleotides in plasma and cellular matrices. Pharm. Res. 26(6): 1251–1264 (2006).
30. Efler SM, Zhang L, Noll BO, Uhlmann E, Davis HL. Quantification of oligodeoxynucleotides in human plasma with a novel hybridization assay offers greatly enhanced sensitivity over capillary gel electrophoresis. Oligonucleotides. 15(2), 119–131 (2005).
31. U.S. Department of Health and Human Services Food and Drug Administration Center for Drug Evaluation and Research (CDER) Center for Veterinary Medicine (CM). Guidance for Industry: Bioanalytical Method Validation. ; 1–22 (2001).