At long last, oligonucleotide drugs are close to deliver on their promise
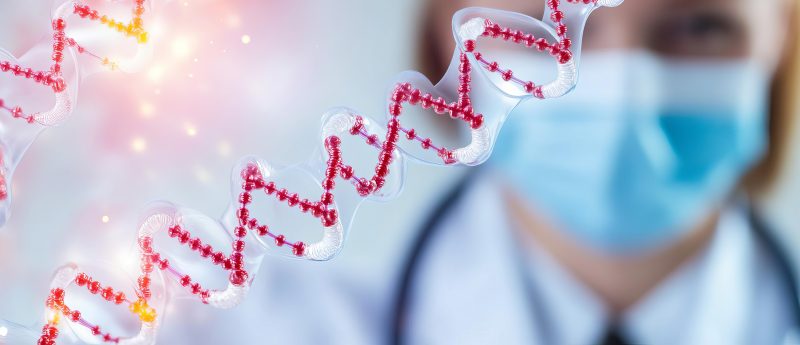
“Recent clinical successes prove that synthetic oligonucleotides are useful therapeutic agents, but some obstacles need to be overcome for a more widespread and safe application.”
About Johannes Winkler
Department of Pharmaceutical Chemistry, University of Vienna (Vienna, Austria), and Department of Cardiology, Medical University of Vienna (Vienna, Austria).Johannes Winklerhas studied pharmacy at the University of Vienna and completed his PhD in pharmaceutical chemistry on development of therapeutic oligonucleotides. He has postdoctoral experience in Austria and Switzerland, investigating nucleic acid chemistry, biology, and the development of targeted drug delivery systems. He has completed his habilitation (venia docendi) for pharmaceutical chemistry in 2014. His research focus is on oligonucleotide chemistry, analysis, biology and pharmacology, regenerative medicine, and nucleic acids biomarkers.
New momentum for oligonucleotide drugs
The promise of exploiting nucleic acids as drug targets using the highly specific, yet modular principle of strand recognition by complementary, artificially-produced oligonucleotides was recognized more than 30 years ago. In particular, the ability of synthetic oligonucleotides to prevent or modulate the translation of practically any gene product based on their base sequence and Watson–Crick base pairing is a great promise for rational targeting and paves the way for personalized medicines. Given their molecular weight of around 6–8 kDa for single stranded agents, oligonucleotide fall somewhere between small molecules and biological macromolecules, which essentially influences their pharmacodynamic and pharmacokinetic properties. Generally based on their pharmacology, oligonucleotides are divided into distinct classes, such as: antisense, siRNA, miRNA mimetics and antagomirs, aptamers and splice-switching compounds. However, since their structural and biophysical properties are shaped by their nucleic acid character, all classes share the main challenges for therapeutic development.
In contrast to traditional drug development, in which the entire process has to start anew for each new target or drug, only the base sequence needs to be properly selected for addressing a particular target. This means that class properties, including stability, tissue distribution and cellular uptake, can be optimized separately and appropriate chemical derivatizations or carrier systems can subsequently be used for virtually any target[1].
Yet, despite intense research and development efforts, it took decades to identify and overcome respective obstacles for enabling application of efficient and safe oligonucleotide therapies. Within the last year the number of marketed oligonucleotide drugs has doubled with the approval of nusinersen and eteplirsen. Mipomersen, pegaptanib, and fomivirsen had been approved earlier, but the latter has been withdrawn for commercial reasons. This clearly gives fresh momentum for this class of therapeutics. Further new drugs, including the first siRNAs, are expected to follow during the next few years. Nevertheless, the new approvals and the ongoing clinical evaluations also highlight the remaining, and so far unsatisfactorily solved, challenges for more widespread, systemic use.
The example of nusinersen
The latest oligonucleotide to be introduced to the market is nusinersen (Spinraza®), a splice-switching agent for treatment of spinal muscular atrophy (SMA)[2,3]. It is the first drug for treating this disease and represents a milestone for both SMA treatment and oligonucleotide technologies. Nusinersen modulates gene splicing of the SMN2 gene and benefits from the high homology between SMN1 and SMN2 genes. In SMA patients, deletions or mutations cause deficiency of SMN1. SMN2 normally produces truncated proteins that are rapidly degraded und thus non-functional, caused by the lack of one exon compared to SMN1.
Nusinersen promotes inclusion of this exon due to binding to the relevant region of the pre-mRNA. Of note, nusinersen is applied by intrathecal administration (lumbar puncture) as oligonucleotides fail to cross the blood–brain barrier. Although a considerable part of the dose is lost to rapid distribution to the systemic circulation, a sufficient concentration remains within the cerebrospinal fluid (CSF), where enough functional SMN protein is then produced to induce significant clinical benefits. The drug is retained in the CSF for a long time, and thus after initial dosing, administration every four months suffices.
Although nusinersen marks a breakthrough for oligonucleotide therapeutics, its success cannot be easily translated to other agents, as it is dependent on the presence of the highly homologous SMN2 gene substituting for the loss of SMN1, and is applied directly to the CSF – the site of action. Thereby, issues related to poor pharmacokinetic properties after systemic application are circumvented.
Other therapeutics are less successful
In late 2016, another splice-modulating oligonucleotide, eteplirsen (Exondys 51 ®), had been approved by the FDA[4]. It is currently under consideration by the EMA for approval in the European Union. Eteplirsen is designed to restore expression of functional dystrophin, the deficiency of which causes muscular dystrophies such as Duchenne muscular dystrophy.
Instead of inducing exon inclusion, it results in exclusion of an exon, but this restores a correct open reading frame that is otherwise lost due to mutations or deletions. Consequently, a protein with higher stability and functionality is produced because the terminal protein domains are essential for linking actin to support proteins. Eteplirsen’s accelerated approval was surrounded by public controversy, as it was based on the surrogate endpoint of enhanced dystrophin in skeletal muscle, which was only a few percent higher than in untreated patients. A clear clinical benefit needs to be proven by further studies.
Due to its chemistry, eteplirsen is rapidly lost by renal elimination, necessitating high and frequent dosing for successful modulation of dystrophin. Furthermore, dystrophin restoration has mainly been proved for skeletal muscle tissue, and it is uncertain whether a therapeutic effect can be induced in heart muscle, which is essential for extending the life expectancy of affected boys.
Mipomersen (Kynamro®) was the first systemically applied oligonucleotide that won approval [5]. It is an antisense agent that targets ApoB-100, a major component of low-density and very low-density lipoprotein, and applied for treating severe familial hypercholesterolemia. Clinical testing has shown consistent increase of liver transaminases as a marker for hepatotoxicity, and a risk evaluation and mitigation plan is enforced. Because of the toxicity concerns, mipomersen was rejected in the European Union, where the market application included weaker forms of familial hypercholesterolemia. The hepatotoxicity is not attributed to the reduction of ApoB-100, but to side effects induced by chemical modifications of the oligonucleotide.
One siRNA that was leading in clinical development, revusiran, was surprisingly withdrawn from a Phase III study in October 2016 after an ad hoc interim analysis found a higher number of deaths in the treatment arm than in the placebo group. Although the cause for toxicity is not fully clear, it may be connected either to the mode of delivery (revusiran uses a glycoprotein receptor in hepatocytes to provide efficient cellular internalization) or to activation of a pattern recognition receptor (Toll-like receptor-3). Other siRNAs and antisense oligonucleotides that use the same hepatocyte receptor uptake mechanism are in clinical development, without reported toxicity issues so far. Unlike antisense and splice-modulating oligonucleotides, only a lower degree of chemical modification is tolerated by siRNAs without losing activity. Nevertheless, modification patterns have been developed that allow sufficient stability for unpackaged siRNAs, and efficient target downregulation and clinical programmes look promising.
Lessons learned for further improvements
These recent examples illustrate the up-and-down journey that oligonucleotides have seen over the past years, and identify the major hurdles and risks; namely adequate biodistribution and cellular uptake, and the potential occurrence of off-target related side effects[6]. In order to ensure sufficient stability against degrading nucleases and functional cellular uptake into the targeted tissue, oligonucleotides need to be either chemically modified or packaged into large carrier systems.
Current solutions in clinical trials are actively or passively targeted at the liver, either by covalent attachment of N-acetyl galactosamine (GalNAc) to oligonucleotides or through encapsulation in liposome-based particles[7]. Successful strategies for modulating extrahepatic targets are still lacking, but are essential in order to fully realize the potential. For ensuring adequate cellular uptake, oligonucleotides must not only enter cells, but also be released from endosomes before being degraded in lysosomes[8]. The basic knowledge of precise uptake pathways seems to be still insufficient for enabling rational design of appropriate carrier systems.
These issues provide a need for respective bioanalytical methodologies. For proving intended therapeutic outcomes, oligonucleotide concentrations should be precisely assessed in blood samples and/or biopsies for establishing pharmacokinetic profiles, as well as directly within targeted cells, preferably in distinct cellular compartments, for ensuring functional delivery. For gathering detailed information, analytical technologies should be able to differentiate between full-length oligonucleotides and shorter metabolites from nuclease digestion. In addition, the downregulation or modulation of the targeted gene in the relevant organ or tissue needs to be proven and linked to a clinical benefit. Off-target activity should be excluded by careful sequence design and selection of appropriate chemical modifications or carrier systems.
During the last decades, important progress in all aspects of pharmaceutical development of oligonucleotide has been made. The pipelines of main companies in the field are well filled with more than 20 individual drugs currently in clinical testing. If the remaining challenges can be overcome and no new safety concerns appear, a rapid increase of approved oligonucleotide drugs will result in the next few years with additional antisense and splice-switching agents entering clinical trials. Several siRNAs are currently in Phase II and III evaluations, market approval of the first siRNA can be expected in 2018 or 2019, and after first successes, additional products will soon follow. Overall, the future looks bright for oligonucleotide therapeutics.
- Khvorova A, Watts JK. The chemical evolution of oligonucleotide therapies of clinical utility. Nat. Biotech, 35(3), 238-248 (2017).
- Wan L, Dreyfuss G. Splicing-Correcting Therapy for SMA. Cell, 170(1), 5 (2017).
- Finkel RS, Chiriboga CA, Vajsar J et al. Treatment of infantile-onset spinal muscular atrophy with nusinersen: a phase 2, open-label, dose-escalation study. The Lancet, 388(10063), 3017-3026 (2016).
- Aartsma-Rus A, Krieg AM. FDA Approves Eteplirsen for Duchenne Muscular Dystrophy: The Next Chapter in the Eteplirsen Saga. Nucl. Acids Therap., 27(1), 1-3 (2016).
- Crooke ST, Geary RS. Clinical pharmacological properties of mipomersen (Kynamro), a second generation antisense inhibitor of apolipoprotein B. Br. J. Clin. Pharmacol., 76(2), 269-276 (2013).
- Dirin M, Winkler J. Influence of diverse chemical modifications on the ADME characteristics and toxicology of antisense oligonucleotides. Expert Opin. Biol. Ther., 13(6), 875-888 (2013).
- Lorenzer C, Dirin M, Winkler A-M, Baumann V, Winkler J. Going beyond the liver: Progress and challenges of targeted delivery of siRNA therapeutics. J. Control. Release, 203, 1-15 (2015).
- Juliano RL. The delivery of therapeutic oligonucleotides. Nucleic Acids Res., 44(14), 6518-6548 (2016).