Insights on the immunogenicity of antibody-drug conjugates
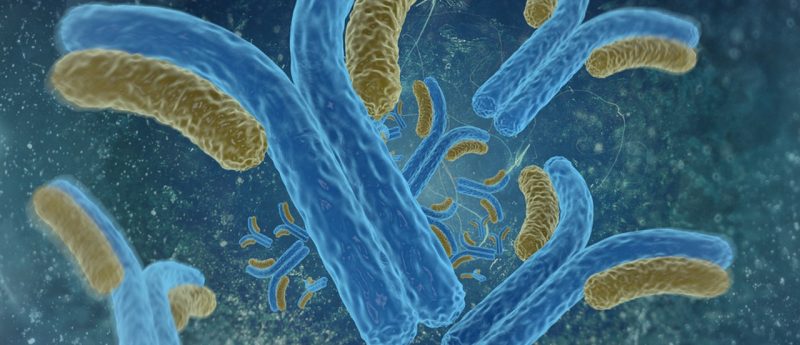
Introduction
Biotherapeutics can elicit an immune response that may alter their pharmacokinetics (PK), efficacy, and safety. Novel biotherapeutics such as antibody-drug conjugates (ADCs) contain chemical domains and have hapten-like structures that may increase their immunogenicity risk.
ADCs are composed of a cytotoxic drug linked to a monoclonal antibody (mAb) which recognizes a tumor-associated antigen. The mechanism of action involves targeted delivery of the cytotoxic drug into cancer cells. Compared to traditional chemotherapeutics, ADCs offer the promise of reduced systemic exposure, lower off-target side effects, and a higher therapeutic index.
Although the concept of ADCs is not new, only two examples are approved for the US market: brentuximab vedotin (Adcetris®, Seattle Genetics) and ado-trastuzumab emtansine (Kadcyla®, Genentech-Roche). Another ADC that gained approval but was later removed from the US market is gentuzumab ozogamicin (Mylotarg®, Pfizer). These ADCs and most of those in clinical development [1] use conventional lysine or cysteine conjugation chemistries that result in a product with heterogeneous drug-to-antibody ratios (DARs), the complexity of which further increases in vivo [2]. Companies are working to make ADCs with reduced DAR heterogeneity, improved conjugate stability and the possibility of an increased therapeutic window [1].
From an immunogenicity perspective, conventional and next-generation ADCs have a hapten-like structure with potentially increased immunogenicity compared to the stand-alone corresponding mAb. The cytotoxic drug component in ADCs is the hapten while the mAb is the carrier. When administered by itself, a hapten is unlikely to induce immunogenicity, but may do so when coupled to a carrier [3]. Humoral immune responses to ADCs can include anti-therapeutic antibodies (ATAs) against all components of the ADC, including the cytotoxic drug, the mAb, and neoepitopes formed in the mAb as a result of the conjugation [3].
ATAs against different domains of an ADC could have different effects on PK, efficacy and safety; this is an important point to consider when developing immunogenicity strategies. Immunogenicity strategies followed for other biotherapeutics are appropriate for ADCs, but with additional characterization to better understand the ATA specificity [4, 5, 6]. These strategies should include a risk-based assessment and assays that are consistent with industry best practices [7].
Immunogenicity risk assessment for ADCs
The immunogenicity risk assessment determines the likelihood that the biotherapeutic will elicit an immunogenic response, and its severity. This likelihood depends on a variety of factors related to both the patient and the product itself [6, 7].
A product-related factor potentially associated with enhanced immunogenicity is the hydrophobicity of the cytotoxic drug. This hydrophobicity could cause the ADC to aggregate, which could cause immunogenicity [6].
Another factor related to the ADC structure is the development of ATAs against the cytotoxic drug. These ATAs could pose a safety risk since uptake of large ADC-ATA immune complexes by non-target immune cells could result in cell death.
Moreover, with many ADCs now in clinical development that use the same linker and cytotoxic drugs as the two approved ADCs, a potential patient-related factor for immunogenicity is prior exposure to a different ADC that contains the same linker or cytotoxic drug on a different mAb.
Because of these unique risks associated with ADCs and limited clinical experience, ADCs should not simply be included in the “low” immunogenicity risk category. However, the risk is generally not perceived as “high” since the immune response would not cause neutralization of an essential endogenous counterpart. Thus, ADCs are generally considered “medium” risk with regards to immunogenicity. However, each ADC may have unique associated risk factors that require careful consideration in the development of specific immunogenicity assay strategies for both nonclinical and clinical studies. In this manuscript I will focus on strategies for clinical studies.
Immunogenicity assay strategies
Immunogenicity evaluation of biotherapeutics such as ADCs requires a set of qualitative and quasi-quantitative assays. A tiered approach that includes screening, confirmatory, and characterization assays can be followed [4, 5].
The first tier is a screening assay, used to determine if the sample is positive or negative for ATAs. The second tier involves confirming the screening positive response, typically by using competitive binding assays with the ADC. The third tier usually involves characterization of ATA domain specificity. Additional characterization may include determination of the relative levels of ATAs (e.g., titering) and determining the neutralizing activity of ATAs. All these immunogenicity assays can be validated following existing guidelines for biotherapeutics [8].
- Screening assays
Bridging assay formats with a homogenous step widely used for other biotherapeutics are well-suited for ADCs. In these assays, the samples are co-incubated with two ADC-labeled reagents followed by immobilization of the ATA-ADC reagent complexes, typically using a streptavidin-coated solid phase. Immunoassays with different readouts are available. Electrochemiluminescence assays (ECLAs) use biotin-ADC and ruthenium-ADC reagents [9,10, 11 &12]. Enzyme-linked immunosorbent assays (ELISAs) with colorimetric readout using biotin-ADC and digoxigenin-ADC reagents and secondary horseradish peroxidase-mouse anti-digoxin detection antibody have been reported [10]. Regardless of the assay platform, test samples with signal responses equal to or above an established threshold are determined as screening positive. These screening assays detect ATAs against the different domains of the ADC and have shown appropriate sensitivity and tolerance to the ADC in the sample.
Preparation of ADC-labeled reagents for homogeneous bridging assays may present some challenges. For example, ADC labeling may be insufficient if the cytotoxic drug and the label compete for the same residues on the antibody. On the other hand, high label-to-ADC ratios may result in epitope masking for ATA detection as well as higher potential for aggregation and poor stability of the ADC reagents.
A challenge for immunogenicity assays is the presence of the biotherapeutic in the sample. An approach to reduce ADC interference consists of selecting sample collection time points at the ADC trough levels. Another approach is to dissociate ATA-ADC immune complexes using acid pre-treatment of the sample.
- Confirmatory Assays
Confirmatory assays typically use the same assay format as the screening assay, with a competitive binding step. The ADC is added to test samples which screened positive. After an incubation period, the spiked and unspiked samples are analyzed with the screening assay. The % signal (e.g., ECLU or absorbance) reduction in the spiked sample compared to the signal in the unspiked sample is calculated. Samples with a % signal reduction equal to or above the established assay threshold are confirmed positive for ATAs.
- Domain Characterization Assays
ATA specificity can be determined against the antibody and linker-cytotoxic drug domains. Two approaches can be followed, competition and direct detection.
In a competition method, samples are incubated with individual unlabeled ADC components (e.g., mAb, linker-cytotoxic drug) and tested using the screening assay format, similar to the confirmatory assay. Reduction of specific signal during competition with ADC components indicates domain specificity [5, 10]. In a direct detection method, specific ADC components are used for capture and/or detection of ATAs against certain domains; appropriately labeled ADC components are required assay reagents [5, 12].
The two methods are capable of detecting the most abundant ATAs but each has different limitations. In the competition approach, it is possible that low abundance ATAs against some ADC epitopes may not be detected in the presence of high abundance ATAs to other epitopes [12]. In the direct detection approach, the molecular structures of the ADC component reagents may differ slightly from the ADC, preventing evaluation of certain immune responses.
Clinical immunogenicity data for ADCs
The limited data on immunogenicity of ADCs come from the two ADCs on the US market and the one discontinued ADC. Additional clinical data are available for other ADCs in clinical development with examples provided elsewhere [5].
Adcetris® used an ECLA to detect ATAs. The ATA incidence was approximately 37%, with all ATAs directed against the chimeric mAb component of the ADC [9]. Neutralizing antibodies were detected in 62% of the ATA-positive patients. Overall, the effect of ATAs on safety and efficacy is unknown. However, infusion reactions were greater in patients with ATAs and in two of the patients (1%) these reactions led to discontinuation of treatment [9].
Kadcyla (T-DM1) used bridging assays in two assay platforms, the Bioveris ECLA technology (later discontinued) and a biotin-digoxigenin based ELISA [10]. ATA specificity was characterized by competitive binding. The overall immunogenicity rate observed was 5.3%, with ATAs primarily against linker-drug and/or neoepitopes in the mAb. No data has been reported on the neutralizing activity of the ATAs. The development of ATAs in patients did not have an obvious impact on the safety, pharmacokinetics and efficacy of T-DM1 compared to patients who tested negative for ATAs to T-DM1 [10].
In the case of Mylotarg, ATAs were not detected in any of the 277 patients enrolled in Phase II clinical studies. Two patients in a Phase I study developed antibodies against the calicheamicin/linker domain. Transient shortness of breath was associated with ATA in one of the Phase I patients [13]. It is possible that ATA responses were not detected since earlier immunogenicity assay formats had low tolerance to the biotherapeutic.
Conclusions and future perspective
In the coming years, additional information is expected from numerous clinical trials with both conventional and next generation ADCs. The new data will provide a broader understanding of the immunogenicity of ADCs and the clinical impact of ATAs against ADCs. We will know if the perceived immunogenicity risk translated in the clinic and perhaps find trends in ATA development and ATA domain specificity for a given ADC platform sharing the same cytotoxic drug, the same linker, and the same drug-to antibody ratio.
There is nothing about ADCs that limits them to oncology. Many therapeutic areas would benefit from a small molecule drug delivered in a targeted way to avoid systemic exposure to a potent drug. Antibody-antibiotic conjugates targeting infectious agents [14] and antibody-glucocorticoid conjugates targeting inflammatory diseases [15] are just two examples being pursued now.
The drive to generate improved ADCs along with the diverse uses will result in many platforms, that is, the combination of small molecule drug, linker, and carrier. Labs are investigating different site-specific conjugations of the linker-cytotoxic drug [16] using engineered natural (e.g., cysteines and selenocysteine) and non-natural (e.g., p‑acetylphenylalanine, p-azido-phenylalanine) amino acids [17]. They are investigating carriers including binding proteins and mAb-derived molecules such as bi-specifics, antibody fragments, and antibody nanoparticle conjugates [17]. These new platforms will introduce more non-natural structures that may increase their immunogenicity.
With such a diverse array of ADCs on the horizon, immunogenicity data from one type of ADC may not help provide general recommendations. The risks associated with the specific small molecules, carriers, and patient populations must be considered to develop the immunogenicity strategies for each ADC.
Note: this editorial is currently in press for publication in Bioanalysis. DOI: 10.4155/BIO.15.86
References:
- Mack F, Ritchie M, Sapra P. The next generation of antibody drug conjugates. Seminars in Oncology. 41(5), 637-652 (2014).
- Shen BQ, Xu K, Liu L et al. Conjugation site modulates the in vivo stability and therapeutic activity of antibody-drug conjugates. Nat. Biotechnol. 30, 184-189 (2012).
- Janeway CA Jr, Travers P, Walport M, Schlomchik M. Immunobiology: the immune system in health and disease (6th edition). Garland Science Publishing, NY, NY (2005).
- Gorovits B, Wakshull E, Pillutla R, Xu Y, Manning MS, Goyal J. Recommendations for the characterization of immunogenicity response to multiple domain biotherapeutics. J. Immunol. Methods. 408(1-12) (2014).
- Hock MB, Thudium KE, Carrasco-Triguero M, Schwabe NF. Immunogenicity of antibody drug conjugates: bioanalytical methods and monitoring strategy for a novel therapeutic modality. AAPS Journal. 17(1), 35-43 (2015).
- Guidance for Industry, Immunogenicity Assessment for Therapeutic Protein Products 2014. Available from: www.fda.gov/downloads/drugs/guidancecomplianceregulatoryinformation/guidances/ucm338856.pdf
- Koren E, Smith HW, Shores E et al. Recommendations on risk-based strategies for detection and characterization of antibodies against biotechnology products. J. Immunol. Methods 333(1-2), 1-9 (2008).
- Shankar G, Devanaravan V, Amaravadi L et al. Recommendations for the validation of immunoassays used for detection of host antibodies against biotechnology products. J. Pharm. Biomed. Anal. 48(5), 1267-1281 (2008).
- Brentuximab vedotin: Summary of Product Characteristics, EMEA 2012. Available from: www.ema.europa.eu/docs/en_GB/document_library/EPAR_-_Product_Information/human/002455/WC500135055.pdf
- Carrasco-Triguero M, Yi JH, Dere R et al. Immunogenicity assays for antibody-drug conjugates: case study with ado-trastuzumab emtansine. Bioanalysis 5 (9), 1007-1023 (2013).
- Carrasco-Triguero M, Mahood C, Milojic-Blair M et al. Overcoming soluble target interference in an anti-therapeutic antibody screening assay for an antibody-drug conjugate therapeutic. Bioanalysis 4(16), 2013-2016 (2012).
- Hoofring SA, Lopez R, Hock MB et al. Immunogenicity testing strategy and bioanalytical assays for antibody-drug conjugates. Bioanalysis 5(9), 1041-1055 (2013).
- Wyeth-Ayerst. Gemtuzumab/Ozogamicin, product insert. Available from: http://www.fda.gov/ohrms/dockets/ac/05/briefing/2005-4191B1_04_02-Wyeth-Insert.pdf
- Yacoby I, Benhar I. Targeted anti bacterial therapy. Infect. Disord. Drug Targets 7(3), 221-229 (2007).
- Graversen JH, Svendsen P, Dagnaes-Hansen F et al. Targeting the hemoglobin scavenger receptor CD163 in macrophages highly increases the anti-inflammatory potency of dexamethasone. Mol. Ther. 20(8), 1550-1558 (2012).
- Panowski S, Bhakta S, Raab H, Polakis P, Junutula JR. Site-specific antibody drug conjugates for cancer therapy. mAbs 6(1), 34-45 (2014).
- Deonarain MP, Yahioglu G, Stamat J, Marklew J. Emerging formats for next-generation antibody drug conjugates. Expert Opin. Drug Discov. 10(5), 463-481 (2015).