Imaging mass spectrometry clinical applications part I: examples from the literature
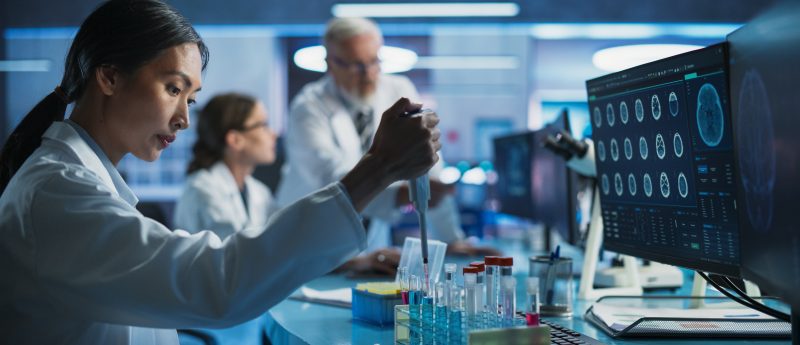
During an interview on National Public Radio’s (DC, USA) Fresh Air, Siddhartha Mukherjee, M.D. credits a single conversation with a cancer patient as the inspiration for his book, The Emperor of All Maladies: A Biography of Cancer. In that exchange, the patient stated very simply, “I’m willing to go on [with further treatment], but before I go on, I need to know what it is I’m battling,” [1]. This simple and honest statement underscores one important reason we seek to understand disease – it gives hope to those combating the disease. As biomedical scientists, we hope that our work will eventually become more than knowledge, that it will lead to improved treatments and patient outcomes.
As a technology MALDI IMS, introduced in 1997 [2], contributes to bridging this gap between knowledge and application. The in situ generation of analyte (e.g., protein, lipid, metabolite) spatial distributions provides the means for reclassification of disease on a molecular level in tissues spanning numerous clinical applications – all with the goal of providing improved patient care. This technology is especially useful to define diagnostic indices where traditional histological methods cannot. In part I of this feature, I will touch upon a few clinical applications. In part II, I will conclude with a recent example from a clinical case.
Tumor staging
Tumors are staged on the basis of location, size, cell type, grade, and degree of metastasis, generally through the use of patient examinations and laboratory and pathology reports [3]. Due to the heterogeneous nature of cancer, these tools are not always sufficient to accurately determine the type or stage of a tumor. Genomics and proteomics are playing an increasing role in diagnostic medicine by providing molecular signatures of disease, facilitating accurate classification and enhanced therapeutic potential [4]. MALDI IMS (including histology-directed profiling) is well suited to this task because it can generate images of analyte distributions in a high-throughput manner without the need for predefined markers.
The first published application of MALDI IMS for tumor staging classified non-small-cell lung cancer (NSCLC) tumors [4,5]. Resected tissues were categorized and staged by pathologists. Subsequent profiling analysis determined molecular signatures from normal, NSCLC tissues, a pulmonary carcinoid, and metastases to the lung, and these were used to build models for tumor class prediction. Classification within a test group of 43 samples for normal versus tumor, normal versus NSCLC, and NSCLC versus other lung tumors was 100 % accurate. Furthermore, NSCLC type was distinguished with 94–100 % accuracy [4,5]. Additional IMS classification studies establish the capability of MALDI IMS for accurate tumor staging [6–9].
Clarification of diagnosis and prognosis
Diseases with similar phenotypes may have different genetic backgrounds or etiologies and may require different therapeutic approaches to reach a successful outcome. Such diseases can be difficult to distinguish histologically and symptomatically. In these cases, molecular profiles that differentiate disease types can lead to proper diagnosis and, importantly, the right treatment approach. The distinction of HER2-positive and HER2-negative breast cancer is a well-known example where the genetic background of the cancer cells will affect the treatment approach [10]. While there are two US FDA approved testing methods for HER2 expression, accurate testing is still a challenge, and MALDI IMS represents a valuable technology for this distinction [11]. In a test set of 18 HER2-positive and -negative tissue samples, MALDI IMS classification models (based on the molecular signature of seven ions) demonstrated 83 % sensitivity and 92 % specificity [11].
Approximately 20% of gastric cancers are also HER2-positive [12]. However, gastric cancers have a high degree of genetic heterogeneity, confounding accurate assessment of HER2 status [13,14]. The MALDI approach described above was applied to breast and gastric cancers to develop an assay for HER2 status independent of tissue type. The resulting proteomics based algorithm achieved an overall classification accuracy of 87 %, with a sensitivity of 78 % and a specificity of 88 %. This example shows the advantages of MALDI IMS over currently approved methods; it is a more efficient, less expensive and, importantly, more objective method to accurately distinguish HER2 status [12].
Beyond diagnosis, disease heterogeneity can make prognosis difficult. For example, stage III metastatic melanoma has an estimated 5-year survival rate that spans from 24–70 % [15]. When applied to a small number of patient samples (n = 86), MALDI IMS was able to classify lymph tissue as normal or metastatic with a 90 % success rate and identified a set of proteins for predicting survival or recurrence [15]. This study demonstrates the utility of MALDI IMS to determine molecular biomarkers for accurate prognosis of disease.
Investigation of drug delivery and efficacy
Monitoring the efficacy of drug delivery and therapeutic response is an important step for improved clinical care. Numerous manuscripts utilizing MALDI IMS to monitor drug delivery and/or efficacy have been published (see reviews [16–19]). The first profiles and images to establish the capability of MALDI IMS in this area came in 1999 [20] and 2003 [21]. In 2013, MALDI IMS was used to gain insight into the mechanisms of fosdevirine toxicity, which was observed in four patients during a Phase IIb clinical trial [22]. Recently, human lung tissue samples from tuberculosis patients treated with a drug cocktail were analyzed by MALDI IMS [23]. Analysis of the resulting drug distributions casts light on the efficacy of these drugs in human tuberculosis lesions, and the outcome of this work may alter treatment approaches to reduce multidrug resistant tuberculosis [23]. The ability of this technology to rapidly [24] and quantitatively [25] detect the spatial distributions of drugs and their metabolites in situ, as well as lipids, peptides, and proteins that could indicate a therapeutic or toxic response, places high value on MALDI IMS for understanding treatment strategies.
MALDI IMS is a powerful technology to enhance our molecular understanding of disease. It complements traditional histological and imaging techniques by providing molecular identifications. Ultimately, diagnostic and prognostic abilities will benefit most when MALDI IMS is used alongside a multimodal imaging suite to improve therapeutic outcomes.
Upcoming Feature:
Imaging Mass Spectrometry Clinical Applications Part II: An example from the clinic, available February 29th.
References
- An oncologist writes “A Biography Of Cancer” [Internet]. NPR.org. (2010). Available from: http://www.npr.org/2010/11/17/131382460/an-oncologist-writes-a-biography-of-cancer.
- Caprioli RM, Farmer TB, Gile J. Molecular imaging of biological samples: localization of peptides and proteins using MALDI-TOF MS. Anal. Chem. 69(23), 4751–4760 (1997).
- Cancer staging [internet]. Natl. Cancer Inst. Available from: http://www.cancer.gov/about-cancer/diagnosis-staging/staging/staging-fact-sheet.
- Chaurand P, Sanders ME, Jensen RA, Caprioli RM. Proteomics in Diagnostic Pathology. Am. J. Pathol. 165(4), 1057–1068 (2004).
- Yanagisawa K, Shyr Y, Xu BJ, et al. Proteomic patterns of tumour subsets in non-small-cell lung cancer. The Lancet. 362(9382), 433–439 (2003).
- Schwartz SA, Weil RJ, Johnson MD, Toms SA, Caprioli RM. Protein profiling in brain tumors using mass spectrometry: feasibility of a new technique for the analysis of protein expression. Clin. Cancer Res. Off. J. Am. Assoc. Cancer Res. 10(3), 981–987 (2004).
- Kang HS, Lee SC, Park YS, et al. Protein and lipid MALDI profiles classify breast cancers according to the intrinsic subtype. BMC Cancer. 11, 465 (2011).
- Morgan TM, Seeley EH, Fadare O, Caprioli RM, Clark PE. Imaging the clear cell renal cell carcinoma proteome. J. Urol. 189(3), 1097–1103 (2013).
- Steurer S, Seddiqi AS, Singer JM, et al. MALDI imaging on tissue microarrays identifies molecular features Associated with Renal Cell Cancer Phenotype. Anticancer Res. 34(5), 2255–2261 (2014).
- How Herceptin works [internet]. Breastcancer.org. Available from: http://www.breastcancer.org/treatment/targeted_therapies/herceptin/how_works.
- Rauser S, Marquardt C, Balluff B, et al. Classification of HER2 receptor status in breast cancer tissues by MALDI imaging mass spectrometry. J. Proteome Res. 9(4), 1854–1863 (2010).
- Balluff B, Elsner M, Kowarsch A, et al. Classification of HER2/neu status in gastric cancer using a breast-cancer derived proteome classifier. J. Proteome Res. 9(12), 6317–6322 (2010).
- Rüschoff J, Hanna W, Bilous M, et al. HER2 testing in gastric cancer: a practical approach. Mod. Pathol. 25(5), 637–650 (2012).
- Treacy AD, Karamchandani JR, Streutker CJ, Grin A. HER2 genetic heterogeneity in gastric cancer: evaluation according to the College of American Pathologists breast cancer criteria. Appl. Immunohistochem. Mol. Morphol. AIMM Off. Publ. Soc. Appl. Immunohistochem. 23(9), 628–632 (2015).
- Hardesty WM, Kelley MC, Mi D, Low RL, Caprioli RM. Protein signatures for survival and recurrence in metastatic melanoma. J. Proteomics. 74(7), 1002–1014 (2011).
- Prideaux B, Stoeckli M. Mass spectrometry imaging for drug distribution studies. J. Proteomics. 75(16), 4999–5013 (2012).
- Ferguson CN, Fowler JWM, Waxer JF, Gatti RA, Loo JA. Mass spectrometry-based tissue imaging of small molecules. Adv. Exp. Med. Biol. 806, 283–299 (2014).
- Greer T, Sturm R, Li L. Mass spectrometry imaging for drugs and metabolites. J. Proteomics. 74(12), 2617–2631 (2011).
- Lietz CB, Gemperline E, Li L. Qualitative and quantitative mass spectrometry imaging of drugs and metabolites. Adv. Drug Deliv. Rev. 65(8), 1074–1085 (2013).
- Troendle FJ, Reddick CD, Yost RA. Detection of pharmaceutical compounds in tissue by matrix-assisted laser desorption/ionization and laser desorption/chemical ionization tandem mass spectrometry with a quadrupole ion trap. J. Am. Soc. Mass Spectrom. 10(12), 1315–1321 (1999).
- Reyzer ML, Hsieh Y, Ng K, Korfmacher WA, Caprioli RM. Direct analysis of drug candidates in tissue by matrix-assisted laser desorption/ionization mass spectrometry. J. Mass Spectrom. JMS. 38(10), 1081–1092 (2003).
- Castellino S, Groseclose MR, Sigafoos J, et al. Central nervous system disposition and metabolism of fosdevirine (GSK2248761), a non-nucleoside reverse transcriptase inhibitor: an LC-MS and matrix-assisted laser desorption/ionization imaging MS investigation into central nervous system toxicity. Chem. Res. Toxicol. 26(2), 241–251 (2013).
- Prideaux B, Via LE, Zimmerman MD, et al. The association between sterilizing activity and drug distribution into tuberculosis lesions. Nat. Med. 21(10), 1223–1227 (2015).
- Prideaux B, Dartois V, Staab D, et al. High-sensitivity MALDI-MRM-MS imaging of moxifloxacin distribution in tuberculosis-infected rabbit lungs and granulomatous lesions. Anal. Chem. 83(6), 2112–2118 (2011).
- Chumbley CW, Reyzer ML, Allen JL, et al. Absolute quantitative MALDI imaging mass spectrometry: a case of rifampicin in liver tissues. Anal. Chem. (DOI: doi: 10.1021/acs.analchem.5b04409) (2016).