Ion mobility spectrometry combined with high-resolution mass spectrometry: acquisition, analysis and application to drug metabolism studies
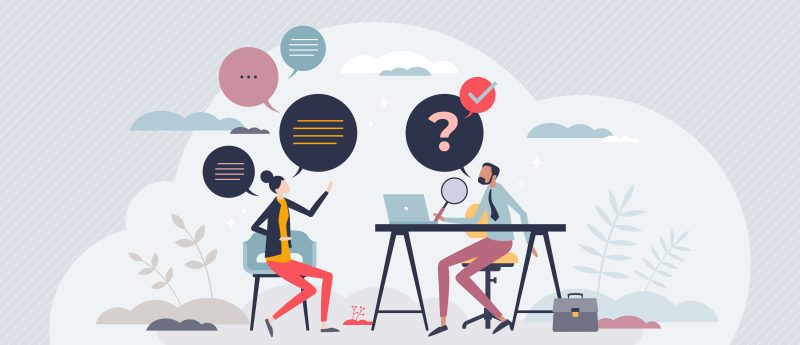
Jan Boerma
Biotransformation Scientist
Unilabs York Bioanalytical SolutionsJan Boerma studied Pharmaceutical Sciences and completed his PhD in Molecular Toxicology at the VU University in Amsterdam (the Netherlands). His doctoral work focused on the use of Glutathione-S-Transferases as tools and targets for the characterisation of chemically reactive drug metabolites. Continuing his interest in drug metabolism he then joined Unilabs York Bioanalytical Solutions, a contract research organisation, in Sandwich (UK) as a Biotransformation Scientist. He is currently performing metabolism studies in support of discovery (e.g. soft spot analysis) and development projects (e.g. radiolabelled ADME and MIST studies).
Dan Weston
Principal Applications Chemist, Mass Spectrometry
Waters CorporationDan Weston has over 20 years experience in mass spectrometry, the majority of which has been gained using LC-MS/MS within the pharmaceutical industry, where he focused on metabolite characterisation for drug discovery (Schering-Plough, USA), drug development and human safety (AstraZeneca, UK). During this time, he also fostered successful collaborations and post-graduate research studentship for complex pharmaceutical analysis using high-resolution mass spectrometry with ion mobility and ambient ionisation techniques. Dan joined Waters in 2014 as a Principal Scientist in the European Applications Laboratory (Wilmslow, UK), where his main roles include driving scientific strategy and value-led collaborations, both across the Waters business and with key industrial peer-groups.
Keywords
Metabolism, ion mobility spectrometry, collision cross section, high-resolution mass spectrometry, biotransformation, metabolite identification
Introduction
Drug metabolism studies continue to play a critical role in the discovery and development of novel drugs. In discovery, the identification of the major routes of biotransformation allows medicinal chemists to design compounds with more desirable absorption, distribution, metabolism and excretion (ADME) properties, whilst identifying metabolite profiles across species aids in the selection of appropriate animal models. In development, radiolabeled studies in humans and animals are conducted to establish definitive metabolite profiles, whilst cross species comparisons of circulating metabolites (MIST studies) provide early insights around metabolite safety [1].
Identification of metabolites across the discovery and development continuum relies on high quality liquid chromatography/mass spectrometry (LC–MS) data. Modern high-resolution mass spectrometers are able to provide rapid, sensitive and accurate information on drugs and their metabolites in a variety of matrices [2]. However, the pressure to reduce the development time and costs for new drugs, which requires feedback of metabolite information earlier in the development process, and the development of increasingly potent drugs require biotransformation scientists to generate more information from samples with low levels of drug-related material. These developments pose an analytical challenge that cannot always be addressed by mass resolution alone. The introduction of commercial ion mobility enabled high-resolution mass spectrometers, in which the drift time of the ions in an ion mobility cell provides an orthogonal dimension of separation, has the potential to address some of these challenges.
Ion mobility separation in data acquisition and analysis workflows
Ion mobility provides separation based upon the size-and-shape of an ion, where smaller more-compact ions traverse the ion mobility cell faster than more-open structures of the same mass-to-charge, prior to high-resolution accurate mass measurement. The time taken to travel through the mobility cell (drift time) is inherently linked to the size-and-shape of the ion and can allow a precise measurement of the overall size, or collision cross section (CCS), in a calibrated system. By integrating routine ion mobility separation and precise CCS measurement in a non-targeted data acquisition – a scan mode referred to as HDMSE, as used on the Waters Vion IMS QTof instrument – the resulting data show improved spectral clarity and aid in more confident metabolite characterization, especially at trace levels. Briefly, this data-independent scan mode relies upon applying alternate low or high collision energies to the mobility-resolved ion population without any precursor ion-selection. As the drift time of the intact precursor and fragment ions are the same, the resulting data functions are aligned automatically using ion mobility to remove unrelated ions, giving simplified precursor (low energy) and aligned fragment ion (high energy) data for easier data interpretation. Secondly, as the measured CCS is largely matrix, concentration and retention time independent, using CCS as an additional parameter for detection and confirmation can bring added confidence. The UNIFI software, which is used for both data acquisition and data interrogation, has an integrated structural algorithm which utilizes conservative, rule-based fragmentation directed via electronic structure (MOL) files within a Scientific Library, for known parent drugs or metabolites. In addition to highlighting in-source fragments and aligning low and high energy data, these structural algorithms combine with automated Discovery Tools to interrogate non-targeted HDMSE data and allow common chemical motifs (e.g. common fragment ions or common neutral losses) to be highlighted for uncharacterized metabolite responses. Together with the crucial input from a skilled biotransformation scientist, these tools provide a seamless approach to compound elucidation whilst greatly reducing the amount of non-value-adding tasks the scientist has to perform when evaluating complex data sets, allowing decisions to be made faster. The data-independent nature of routine HDMSE acquisition also ensures a degree of future-proofing, meaning that as the scientific question changes, these legacy non-targeted HDMSE data remain relevant [3,4].
Application of ion mobility separation in discovery and development metabolism projects
During early drug discovery, large numbers of compounds are assayed in liver microsomes and hepatocytes to determine metabolic clearance and the major biotransformation products (soft spot analysis). Generic chromatography methods, using short run times and rapid gradients, and acquisition of conventional accurate-mass high-resolution mass spectrometric data are generally used in order to analyze the large number of samples in a timely manner. Such chromatographic conditions regularly result in the (partial) co-elution of drug-related components, which impedes the structural elucidation of metabolites from untargeted mass spectrometric data and could result in incorrect structural assignments. Consequently these samples may require reanalysis using targeted fragmentation of precursor ions for confident characterization, delaying the feedback of metabolite information. However, provided that co-eluting metabolites have sufficiently different drift times, these components can be resolved using HDMSE acquisition on ion mobility enabled high-resolution mass spectrometers, resulting in component-specific fragmentation data and rapid structural elucidation of the metabolites involved [4].
In addition to metabolite co-elution, the complex biological matrices (e.g. bile and plasma) resulting from in vivo discovery metabolism studies hinder metabolite elucidation using conventional non-targeted acquisition of mass spectrometric data due to the large number and high abundance of fragment ions from endogenous components. In workflows using HDMSE the endogenous components can often be largely resolved from drug-related components by ion mobility separation, resulting in metabolite precursor and fragment ion spectra with less interference from endogenous components [5].
Radiolabeled ADME studies, which are performed during the development phase, also involve the analysis of complex matrices (i.e. urine, faeces and plasma). These metabolism studies can be further complicated by low metabolite levels resulting from administration of highly potent drugs. The increased quality in mass spectrometric data provided by ion mobility separation following HDMSE acquisition is particularly useful when sample availability is limited, for instance for plasma with low levels of drug-related material, such that all metabolite information must be generated from a single analysis.
The precise measurement of CCS values for all drug-related components using ion mobility allows metabolites to be tracked across matrices analyzed under identical chromatographic conditions, where retention time shifts can occur as a consequence of matrix effects, and it offers the possibility for metabolite identities to be compared across the varying chromatographic conditions used during drug discovery and development projects [3].
Conclusion
The identification of drug metabolites, which is a key element of drug discovery and development programs, depends on high quality mass spectrometric data. The increasing pressure for rapid and robust metabolite identification in discovery and the challenge to provide metabolite information for highly potent drugs in complex matrices in development programs can show the limitations of mass-resolution alone. Some of these limitations can be addressed by ion mobility enabled high-resolution mass spectrometers, such as the Waters Vion IMS QTof, in which ion mobility provides an additional dimension of separation. The routine and robust acquisition of ion mobility data improves the quality of the mass spectra for drug-related components and generates information on the size-and-shape of the components (i.e. CCS), allowing drug metabolism scientists to identify metabolites faster and with more confidence.
References
[1] Drug Metabolism in Drug Design and Development: Basic Concepts and Practice. Zhang D, Zhu M and Humphreys WG. John Wiley & Sons, Inc. (2008).
[2] Wen B, Zhu M. Applications of mass spectrometry in drug metabolism: 50 years of progress. Drug Metab. Rev. 47(1), 71–87 (2015).
[3] Holdsworth C et al. Utilization of ion mobility enabled collisional cross section measurements for the comparison of metabolites across differing chromatographic methods. Presented at the DMDG open meeting 2016. http://www.waters.com/waters/library.htm?cid=511436&lid=134917233
[4] Savage M et al. Can the routine use of ion mobility in drug metabolism studies aid with the efficient and robust characterisation of drugs and their metabolites? Presented at the DMDG open meeting 2017. https://www.bioanalysis-zone.com/2017/10/09/ion-mobility-drug-metabolism-studies-aid-efficient-robust-characterisation-drugs-metabolites_spotl_emerging_tech_waters/
[5] Answering Critical Questions in Biotransformation using Mass Spectrometry with Routine Ion Mobility. Weston D, Boerma J. Webinar Bioanalysis Zone 2017.
https://www.bioanalysis-zone.com/2017/09/20/answering-critical-questions-in-biotransformation-using-mass-spectrometry-with-routine-ion-mobility_waters/
Our expert opinion collection provides you with in-depth articles written by authors from across the field of bioanalysis. Our expert opinions are perfect for those wanting a comprehensive, written review of a topic or looking for perspective pieces from our regular contributors.
See an article that catches your eye? Read any of our Expert Opinions for free.