The single cell proteomics revolution
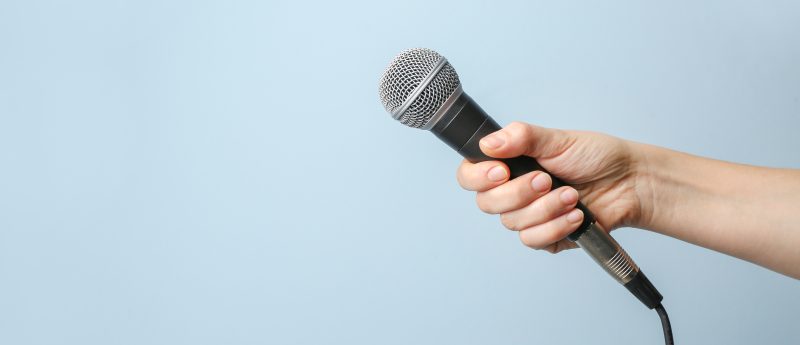
Ben Orsburn received his PhD at Virginia Tech (VA, USA) by applying mass spectrometry to solve biological problems. He has held positions at Johns Hopkins (MD, USA), Thermo Fisher Scientific (CA, USA), NIAID (MD, USA) and the National Cancer Institute (MD, USA). He has spent time developing LC–MS methods in over 150 labs around the world and utilizes these skills as the founder of LCMSmethods.org, a volunteer organization that provides validated instrument methods to researchers in an increasingly broad range of application areas. This exclusive editorial explores the very recent and exciting developments in the field of single cell proteomics....