Robert MacNeill: Balancing instability precautions against risk of adsorptive losses
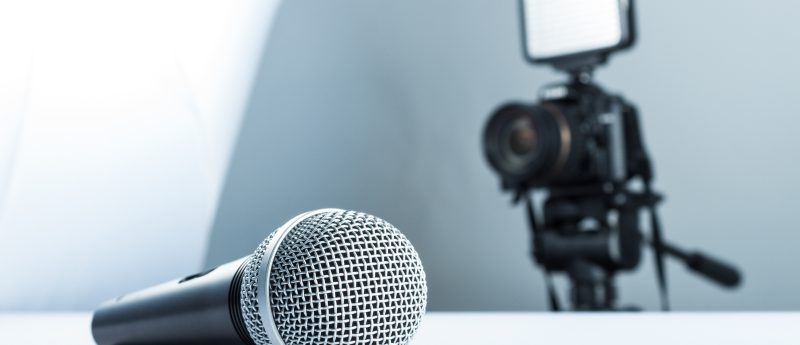
In this installment of Robert MacNeill’s (Envigo) column, Robert discusses the instability of drugs within samples and how to balance their instability against absorptive loss.
Robert MacNeill received his Bachelor’s degree with Honors in Chemistry from Heriot Watt University then his MSc in Analytical Chemistry from the University of Huddersfield, both in the United Kingdom. Robert is also a Chartered Chemist and a Fellow of the Royal Society of Chemistry. With 20 years of experience in all aspects of quantitative bioanalytical LC–MS/MS method development, 11 of these years heading method development activities within HLS/Envigo, and a regular author and peer reviewer for the journal Bioanalysis, Robert is a recognized expert and innovator in the field.
The instability of drugs, drug candidates, metabolites or any designated analyte within samples of a biological nature is a very familiar topic of discussion in the regulated bioanalytical community. Indeed, the guidance and typical standard operating procedures are now such that any significant degree of instability will be confidently detected and dealt with. It is my impression, however, that the exposure of the community to so much rhetoric and discussion concerning instability issues has led to something of a blind spot regarding the ramifications of some routine aspects of bioanalytical work that may be affected by the nature of instability precautions.
I am alluding to two areas primarily. Firstly, the ubiquitous preference to default to storage of samples, stock solutions and spiking solutions at as low a temperature as is available. This is typically -80°C. Secondly, the preference to prepare and extract samples ‘on ice’ in situations where instability is anticipated or at least suspected.
Prevalent on my mind is an effect which is related to the above in its more severe manifestation at lower temperatures. Like instability, it can also lead to pronounced and variable losses, and can very well tear a method’s reliability to shreds. Also, it is probably better-known to those who have worked much in quantification of larger biomolecules. It is the phenomenon of non-specific adsorption (NSA), also known as non-specific binding, and it certainly merits an important influence on the decision as regards sample treatment, including storage, in all appropriate media. It manifests through chemical interactions, ranging from electrostatic to van der Waal’s in nature, resulting in adsorption of compounds from their liquid medium to surfaces that the medium is in contact with.
This article is part of Robert MacNeill’s (Envigo) quarterly column for Bioanalysis Zone which focuses on quantitative method design. You can read past installments of the column here.
There are aspects of the phenomena that are critical to understanding how it works and to making effective decisions in light of it. These factors are the basic mechanism, the concentration (not just of test items but also of other components in the medium), the composition of said liquid medium, the chemistry of the inner surface of the containing vessel, which meets the liquid stored, and the temperature.
From a chemistry perspective, in simple terms, a polar compound will bind to a polar surface, like glass. A nonpolar compound will bind to a nonpolar surface. Chromatographers will be none too unfamiliar with this kind of reasoning. There are two common vessel compositions in the laboratory – glass and polypropylene. To avoid NSA, it makes sense to use glass vessels to contain nonpolar compounds, and polypropylene vessels to store polar compounds, particularly when there are doubts over the free solubility of test compounds in the liquid medium. There are specialty tubes and 96-well plates commercially available that can help, such as polypropylene plates with a glass-like coating.
There is concentration dependence in that the lower the concentration, the more significant any analyte loss will be, as a percentage. This is down to there being a defined number of adsorptive sites, a set surface area available in any given situation. Tying in with this is the effect of the presence in solution of other components. These can alleviate NSA by their own occupation of adsorptive sites, the competition aspect within the solvation and adsorption dynamics. This is why solutions can be particularly susceptible to being affected by NSA, whereas a biological matrix such as plasma, containing a host of different components, may not be as adversely affected. On this note, urine samples in polypropylene tubes may often suffer from the effects of hydrophobic NSA of analyte due to a distinct lack of protein and lipid content compared to plasma. Exacerbating this is the pronounced content of highly water-soluble components in urine, making it all the more difficult for a hydrophobic analyte to remain freely solubilized. Indeed the composition of the liquid medium is key to the manifestation, or otherwise, of NSA. This relationship is also pretty simple. The more soluble a compound is in a certain solution composition, the less chance there is of NSA to surrounding surfaces. If solubility is sparing, the risk of adsorptive losses is always there. Also, the way solubility works is that as the temperature drops, solubility also decreases. This increases the chances and abundance of NSA to the surrounding surfaces, whatever the chemistry.
Temperature is the pivotal point in this discussion. As just stated, NSA is intimately related to solubility in that the lower the temperature, the more severe the NSA. Therefore, wherever temperature can be made closer to ambient without instability or evaporative concerns, the better. Now, if the intriguing reality of matrix aging was not a concern for many types of stored biological samples, from the NSA avoidance perspective when -20°C can be used instead of -80°C, it should be used. Similarly, analyte-containing solutions could often be stored at 4°C instead of at more severe lower temperatures, where later resolubilization of subsequently bound analyte could be difficult and impactful on concentration data. This applies especially if the analytes involved are well-characterized and stability is established.
When working to prepare samples ‘on ice’, meaning in an ice-water bath, the chances of NSA increase due to the lowering of temperature. This is something that is clearly preferable to avoid if it may be feasible to do so, and for this reason it is recommended to work prior to screen for and optimize the best stabilizing additive, at ambient temperature, as an alternative. Another reason to avoid this scenario is that the temperature is harder to control compared to the ambient temperature climate-controlled laboratory, so the ‘on ice’ situation would lend variability to the procedure in this way as well.
The outcome, fortunately, is such that there is not much complexity to make decisions around. Safeguard against NSA principally by selecting storage vessels that the test item has minimal propensity to adsorb to, and where possible by choosing a medium in which the compound’s solubility is maximized. Additionally, it may often be wise to use not quite as low a temperature for storage if there is enough confidence about stability. When faced with the prospect of working ‘on ice’, take the time to investigate if the right stabilizer additive at the right concentration can negate this requirement, and a room temperature operation can proceed.
Our expert opinion collection provides you with in-depth articles written by authors from across the field of bioanalysis. Our expert opinions are perfect for those wanting a comprehensive, written review of a topic or looking for perspective pieces from our regular contributors.
See an article that catches your eye? Read any of our Expert Opinions for free.