Biomarker discovery by mass spectrometry: the potential for API techniques
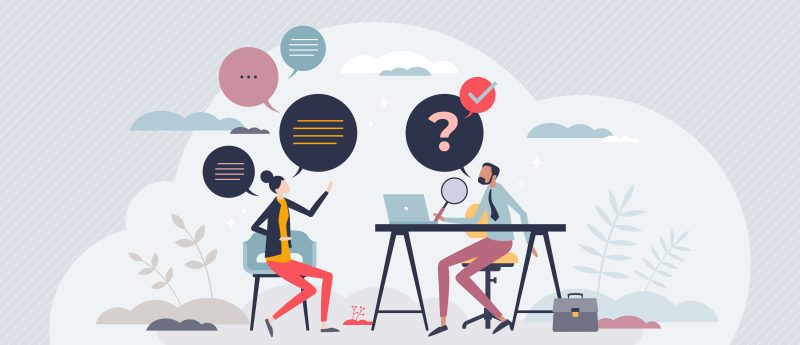
Stephanie Rankin-Turner
Dr Stephanie Rankin-Turner is a Postdoctoral Research Associate in the School of Sport, Exercise and Health Sciences at Loughborough University in the UK. She is currently working on a Partnership for Clean Competition-funded project in the labs of Dr Liam Heaney and Dr Owen Davies. Stephanie graduated with an MSci in Forensic Science from the University of Kent (UK) and a PhD in Analytical Chemistry from Loughborough University. Her PhD research focused on the development of atmospheric pressure ionization mass spectrometry techniques for the direct analysis of human biological fluids, specifically for the differentiation and age estimation of biofluids for forensic purposes. Her current research involves the development and application of mass spectrometry techniques to anti-doping in sports science.
Liam M Heaney
Dr Liam Heaney is a Lecturer in Bioanalytical Science in the School of Sport, Exercise and Health Sciences at Loughborough University. He has research interests in high-throughput measurement of small molecule metabolite biomarkers. This includes actively working on projects that span across multiple disciplines including clinical diagnostic and prognostic applications, sports anti-doping analyzes and biomonitoring of exercise performance/fatigue. His work is centered around the application of analytical chemistry applying both gas chromatography and liquid chromatography-mass spectrometry. Previously, Liam studied an undergraduate degree in Sport and Exercise Science at Loughborough University, where he continued to a Masters in Exercise Physiology and a PhD in Analytical Chemistry (mass spectrometry). Prior to his current position, Liam spent time as a postdoctoral researcher at the University of Leicester’s NIHR Cardiovascular Biomedical Research Centre where he specialized in the analysis of cardiovascular disease biomarkers using mass spectrometry-based techniques.
For many diseases, survival rates are highly dependent on early diagnosis and the administration of effective treatments. Early detection can aid clinical decision making, permit faster treatment and ultimately improve clinical outcomes. Diagnostic tests are often based on the measurement of a biological marker, or biomarker, a quantifiable chemical component, which alters in response to a change in the body. These biomarkers may be characteristic of a disease, such as cancer or cardiovascular disease, or exposure, for example to a hazardous substance. In recent years, a great deal of research has been conducted in the identification of novel biomarkers measured in biofluid and tissues samples. These have been investigated for applications such as indicating disease presence or assisting in patient risk management. Biomarker discovery aims to identify markers associated with specific diseases for the development of laboratory tests to aid in early diagnosis and inform therapeutic interventions to enhance patient survival and quality of life.
Mass spectrometry (MS) has been a gold standard measurement technique in research laboratories for decades and in recent years has proven instrumental in the discovery of new and potential biomarkers. MS-based biomarker discovery often begins with untargeted studies incorporating samples from diseased and healthy patients. By conducting large-scale ’omics studies (e.g. metabolomics), typically using chemometrics to compare the hundreds or thousands of metabolites simultaneously measured, metabolic differences between patient groups can be established and relevant biomarkers identified by pinpointing components unique to diseased patients. The discovery of new biomarkers necessitates extensive studies incorporating hundreds if not thousands of samples, inevitably requiring a significant investment in time for both sample and data analysis. Many studies have reported potential markers of different diseases; however, few have demonstrated real clinical impact. The discovery of new biomarkers not only involves the initial identification of a candidate analyte, but also the extensive validation of the marker and the relevant methods of detection prior to implementation in a clinical setting.
The time-consuming processes in biomarker discovery are major limiting factors. Despite the widespread application of MS in this area, traditional techniques are subject to a number of limitations that can hinder their ability to achieve high-throughput analysis. A major bottleneck in MS analysis is the sample pretreatment required prior to analysis, particularly in the case of complex biological matrices such as blood and urine. These labor-intensive and time-consuming processes often include analyte extraction, purification and chromatographic separation prior to MS analysis. In addition, conventional instruments typically have a large footprint and necessitate high-vacuum systems, confining them to the lab bench and thus requiring the transportation of samples for analysis, inevitably increasing the time taken to obtain a result and elevating the potential for sample degradation. Therefore, the development of biomarker testing using compact or transportable mass spectrometers would enable rapid assessment for point-of-care testing in clinics, reducing potentially lengthy lead times to inform treatments that could save lives.
In recent years, an array of atmospheric pressure ionization (API)-MS techniques have been developed to resolve some of the limitations associated with traditional technologies, enabling the direct analysis of samples in their native state. With minimal or no sample preparation, and no chromatographic separation, such techniques offer a drastically reduced sample analysis time, offering the potential to accelerate both initial biomarker discovery and biomarker detection for clinical investigations. Furthermore, without the need for vacuum systems, atmospheric ion sources are amenable to in situ analysis, opening up the possibility of rapid point-of-care testing. Traditional MS techniques also require a degree of expertise in the pretreatment of samples, use of analytical instruments and interpretation of data. However, API techniques demonstrate great potential for use by the non-expert. This has already been demonstrated with the iKnife, a surgical knife coupled with a mass spectrometer. The handheld surgical tool analyses ‘smoke’ from tissue cauterised upon contact during surgery, providing surgeons with a simple, real-time confirmation of whether they are cutting healthy or cancerous tissue. This is achieved by using a prebuilt database of mass spectra from healthy and cancerous tissue for comparison [1]. This technology is already being assessed in clinical trials [2].
Numerous API techniques have been developed that show great promise for the fast and direct measurement of molecules in biological samples. Matrix-assisted laser desorption ionization (MALDI) has proven to be a powerful tool for imaging materials such as biological tissues, particularly in the study of healthy and cancerous tissue. In MALDI, the sample surface is coated with a matrix solution and a laser is moved and fired across the material, allowing subsequent desorption and ionization of analytes. MALDI has been applied to the analysis of lung cancer [3], colorectal tumor [4], and gastric cancer tissues [5], among others. Proteins, in particular, are the target of many biomarker discovery studies, traditionally analyzed by LC–MS/MS following enzymatic digestion. However, MALDI-MS enables the rapid analysis of proteins from intact tissue samples with minimal sample preparation. Furthermore, with the imaging capabilities offered by MALDI-MS, not only is it possible to pinpoint the chemical differences in cancerous tissue and thus cancer-associated biomarkers, but also build an image of healthy and diseased tissue. Advancements have also been achieved in the surface desorption of liquid-based samples. For example, liquid atmospheric pressure MALDI has provided additional avenues to investigate biofluids for MALDI-MS profiling of diseases [6]. Additionally, liquid extraction surface analysis, or LESA, combines a micro-liquid extraction of a solid-state sample coupled with nano-electrospray ionization MS [7]. This technique has already been successfully applied to the analysis of dried blood spots [8], as well as the assessment of tissue slices for lipid and protein content [9,10].
Probe electrospray ionization (PESI) consists of a solid needle, the end of which is briefly touched to the surface of a sample, enabling a small amount of liquid to be picked up. The needle is positioned in front of a mass spectrometer inlet and a high voltage is applied to induce electrospray, offering an overall sampling and analysis time of just a few seconds per sample. PESI-based techniques have been demonstrated in the rapid and direct analysis of biological materials, including discovery-based metabolomics for plasma biomarkers of atherosclerosis [11] and human tissues (e.g. breast cancer) [12]. This technique enables the detection of a range of molecules, from low molecular weight components to large proteins, and would be readily applicable to the rapid measurement of biological materials for biomarker detection. Adaptations to the PESI source have also allowed the rapid analysis of dried or solid samples. This has been performed using a supply of solvent within a capillary surrounding the PESI needle, extracting the analytes and allowing measurement following a similar approach to PESI-MS [13]. This approach has not yet been applied in a clinical research setting, but recent work has demonstrated its suitability for multiple biofluid assessments [14].
Paper spray ionization has also shown great promise in biological fluid analysis. This is a simple, cost-effective technique, which utilises a small triangular segment of filter paper onto which the sample and a small amount of solvent is applied. As a high voltage is applied to the paper, analyte molecules within the sample are desorbed and ionized from the tip of the triangle, immediately drawn into the mass spectrometer for analysis [15]. Paper spray enables the preparation-free analysis of biological samples and has been proven to be successful in the analysis of blood [15,16],urine [17], and biological tissues [18]. Furthermore, paper spray ionization has been successfully coupled with portable mass spectrometers, demonstrating its potential in point-of-care testing for diagnostics [19].
The use of atmospheric pressure ionization MS in biomarker detection is still in its infancy and extensive research and validation of methods would be required to ensure the development of robust, reproducible methods. Whilst all of the discussed methods demonstrate excellent potential for clinical biomarker analyses, the true potential of API techniques has not yet been realised. However, with the possibility of preparation-free, high-throughput sample analysis and the potential for use with portable mass analyzers, the use of API technology could truly revolutionize biomarker discovery and point-of-care measurements.
References
1. Schäfer K-C, Dénes J, Albrecht K et al. In vivo, in situ tissue analysis using rapid evaporative ionization mass spectrometry. Angew Chemie Int Ed. 48(44), 8240–8242 (2009).
2. Vaqas B, Cameron SJ, Alexander JL, O’Neill KS, Kinross JM, Takats Z. The iKnife: development and clinical applications of rapid evaporative ionization mass spectrometry. In: The Handbook of Metabolic Phenotyping. Lindon JC, Nicholson JK, Holmes E (Eds) Elsevier, Amsterdam 219–236 (2019).
3. Rahman SMJ, Gonzalez AL, Li M et al. Lung cancer diagnosis from proteomic analysis of preinvasive lesions. Cancer Res. 71(8), 3009–3017 (2011).
4. Hiraide T, Ikegami K, Sakaguchi T et al. Accumulation of arachidonic acid-containing phosphatidylinositol at the outer edge of colorectal cancer. Sci Rep. 6(1), 29935 (2016).
5. Balluff B, Rauser S, Meding S et al. MALDI imaging identifies prognostic seven-protein signature of novel tissue markers in intestinal-type gastric cancer. Am. J. Pathol. 179(6), 2720–2729 (2011).
6. Hale OJ, Morris M, Jones B, Reynolds CK, Cramer R. Liquid atmospheric pressure matrix-assisted laser desorption/ionization mass spectrometry adds enhanced functionalities to MALDI MS profiling for disease diagnostics. ACS Omega. 4(7), 12759–12765 (2019).
7. Eikel D, Vavrek M, Smith S et al. Liquid extraction surface analysis mass spectrometry (LESA-MS) as a novel profiling tool for drug distribution and metabolism analysis: the terfenadine example. Rapid Commun Mass Spectrom. 25(23), 3587–3596 (2011).
8. Griffiths RL, Dexter A, Creese AJ, Cooper HJ. Liquid extraction surface analysis field asymmetric waveform ion mobility spectrometry mass spectrometry for the analysis of dried blood spots. Analyst. 140(20), 6879–6885 (2015).
9. Hall Z, Chu Y, Griffin JL. Liquid extraction surface analysis mass spectrometry method for identifying the presence and severity of nonalcoholic fatty liver disease. Anal Chem. 89(9), 5161–5170 (2017).
10. Havlikova J, Randall EC, Griffiths RL et al. Quantitative imaging of proteins in tissue by stable isotope labeled mimetic liquid extraction surface analysis mass spectrometry. Anal Chem. 91(22), 14198–14202 (2019).
11. Johno H, Yoshimura K, Mori Y et al. Detection of potential new biomarkers of atherosclerosis by probe electrospray ionization mass spectrometry. Metabolomics 14(4), 38 (2018).
12. Mandal MK, Chen LC, Hiraoka K. Sequential and exhaustive ionization of analytes with different surface activity by probe electrospray ionization. J. Am. Soc. Mass Spectrom. 22(9), 1493–1500 (2011).
13. Rahman MO, Mandal MK, Shida Y et al. Development of sheath-flow probe electrospray ionization (SF-PESI). J. Mass Spectrom. 48(7), 823–829 (2013).
14. Rankin-Turner S, Ninomiya S, Reynolds JC, Hiraoka K. Sheath-flow probe electrospray ionization (sfPESI) mass spectrometry for the rapid forensic analysis of human body fluids. Anal Methods. 11(29), 3633–3640 (2019).
15. Wang H, Liu J, Cooks RG, Ouyang Z. Paper spray for direct analysis of complex mixtures using mass spectrometry. Angew Chemie Int Ed. 49(5), 877–880 (2010).
16. Manicke NE, Abu-Rabie P, Spooner N, Ouyang Z, Cooks RG. Quantitative analysis of therapeutic drugs in dried blood spot samples by paper spray mass spectrometry: an avenue to therapeutic drug monitoring. J. Am. Soc. Mass Spectrom. 22(9), 1501–1507 (2011).
17. Naccarato A, Moretti S, Sindona G, Tagarelli A. Identification and assay of underivatized urinary acylcarnitines by paper spray tandem mass spectrometry. Anal Bioanal. Chem. 405(25), 8267–8276 (2013).
18. Wang H, Manicke NE, Yang Q et al. Direct analysis of biological tissue by paper spray mass spectrometry. Anal Chem. 83(4), 1197–1201 (2011).
19. Silva LC da, Pereira I, Carvalho TC de, Allochio Filho JF, Romão W, Gontijo Vaz B. Paper spray ionization and portable mass spectrometers: a review. Anal Methods. 11(8), 999–1013 (2019).
The opinions expressed in this feature are those of the author and do not necessarily reflect the views of Bioanalysis Zone or Future Science Group.
Our expert opinion collection provides you with in-depth articles written by authors from across the field of bioanalysis. Our expert opinions are perfect for those wanting a comprehensive, written review of a topic or looking for perspective pieces from our regular contributors.
See an article that catches your eye? Read any of our Expert Opinions for free.