Interview with Erin Chambers (Waters Corporation) on overcoming matrix effects in large molecule bioanalysis
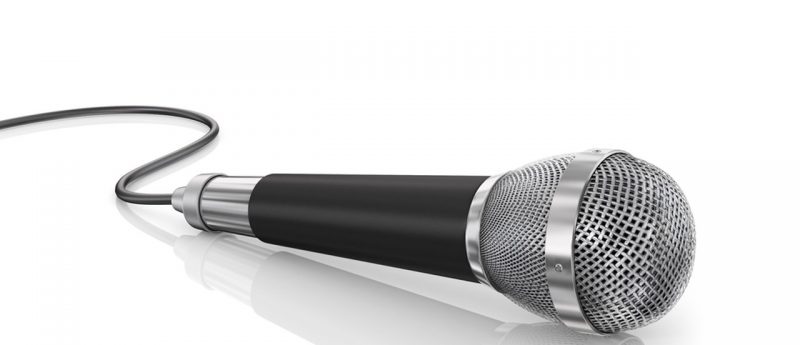
Erin received her B.S. in Chemistry from Yale University. She began her career as an Analytical Chemist at Uniroyal Chemical where she developed analytical methods to determine pesticide metabolism in plants. As a PK/PD scientist at Pfizer, she conducted PK/PD studies in the Animal Health Safety and Metabolism group. This included IV and oral dosing, venapuncture, sample extraction method development, in vivo and in vitro studies, LC/MS/MS analysis, method development, determination and reporting of PK parameters. Erin held positions in Service and R&D at Applied Biosystems before joining Waters Corporation in 2000 as a Proteomics Demo Chemist. In this position she performed QTOF/nano-LC and MALDI demos and provided customer training on same at Micromass. In her current position as Principal Applications Chemist, she provides pharmaceutical applications development and support for regulated and discovery bioanalysis and develop bioanalytical methods for drug molecules (large and small) in human and animal biological matrices. Erin manages the bioanalysis and clinical applications development group and provides customer and in-house training on MS, LC, sample preparation and bioanalysis. After 19 years experience in both large and small molecule mass spectrometry and bioanalytical method development, her most recent focus has been on peptide and protein bioanalysis.
Could you tell Bioanalysis Zone a little about your current role at Waters Corporation?
I am a Principal Applications Chemist in the Chemistry division. I develop all sorts of bioanalytical applications primarily focusing on large molecules. I also do internal and external training and support.
You have recently developed several bioanalytical methods for peptides in both human plasma and cerebrospinal fluid. In general, what are the challenges you have faced when analyzing peptides?
Quantifying peptides for bioanalytical purposes has been the primary focus of my lab over the past 6 or 7 years. We have devised a list of top challenges or problems associated with developing bioanalytical methods for peptides. Some of the highest probability issues are non-specific binding, sensitivity challenges, issues associated with protein binding, aggregation, solubility, specificity and carryover.
In some of the applications that we have developed more recently, we are looking at large peptides like insulin, a peptide called teriparatide and amyloid beta peptides (biomarkers of Alzheimer’s). These challenging large peptides exemplify a lot of the issues and challenges of large-molecule bioanalysis, and have allowed us to learn a lot about how to deal with these problems. This has also taught us how to look at a peptide sequence or properties and predict which challenges that peptide will have before method development starts so that we can put in place some precautionary measures.
Perhaps one of our most successful methodologies has been the quantification of amyloid beta peptides in cerebrospinal fluid. This was where we initially got a sense of the level of difficulty associated with the analysis of peptides of this type, especially in contrast to bioanalysis of small molecules. Many of us, including myself, who have been in bioanalysis for over 20 years, base most of our experiences around the bioanalysis of small molecules. The transition into quantifying these larger molecules can be a difficult one, unless you really know what to expect and how to address the various problems. Working on the amyloid beta project, we were faced with very high levels of non-specific binding, low levels present in cerebrospinal fluid, dealing with protein binding and aggregation and maintaining solubility throughout, not just through the extraction process but also through the injection process.
What strategies did you put into place to overcome these challenges?
There are some very interesting analytical strategies we put into place to deal with these issues. The first was to realize that anytime you are working with solvent standards, there needs to be some carrier protein present in order to minimize non-specific binding to collection plates or vials, especially non-specific binding that would occur in the LC and throughout the chromatographic process. This knowledge was something that we carried over to dealing with teriparatide and insulin peptides. This holds true for solvent standard analysis and it also holds true when working with some of the less fatty/protein laden matrices like cerebrospinal fluid or urine. Typically, once you get into working in a biological matrix, non-specific binding is less likely. For example, if you are working in plasma or serum then there are enough proteins, lipids and other molecules of that nature floating around in the extract that can minimize non-specific binding.
Another challenge that we ran into with the amyloid beta peptides was aggregation and obtaining a true accurate quantitative measure of total amyloid beta (1-42 in particular) in cerebrospinal fluid. A true measure of total abeta 1-42 is critical as it pertains to its use as a biomarker. Many ligand binding assays actually measure free only. To solve this we included a guanidine hydrochloride denaturation step in the initial cerebrospinal fluid pretreatment, followed by an appropriate dilution to put the analyte in the right charge state for subsequent solid-phase extraction. The pre-treatment and extraction processes we developed for these amyloid beta peptides have now become part of a methodology that is the subject of a global effort to standardize a reference method for amyloid beta 1-42. It is very exciting to have your work translating into a potential reference method!
We have had to pull out all the stops in terms of maximizing solubility and minimizing carryover for some of these larger peptides. The more we work with peptides of this type, the more we realize that the strategies tend to be the same. We are doing things such as decreasing LC flow rate, increasing column temperature, and using column chemistries with a stationary phase that is modified with a positively charged surface. It really becomes important when you try and put into place methodologies that may have worked well for small molecules and all of a sudden you are finding issues with carryover, ghost peaks and poor peak shape/loss of peaks entirely. You then really need to start looking at the method development in a different manner. This is where simple solutions such as flow rate adjustment, shallowing of the gradient, increasing temperature or changing column chemistry become critical to getting the performance that you need, whereas with small molecules there is a lot of flexibility and it is very forgiving.
How has technology evolved in recent years in terms of minimizing matrix effects?
I think two of the key technology advancements are the use of these mixed-mode solid phase extraction (SPE) sorbents, as well as the advent and widespread implementation of sub 2-micron LC.
Mixed mode SPE is probably one of the single most effective types of sample preparation, with the exception of maybe affinity, at reducing or minimizing matrix effects. It can do that through dual retention mechanisms, they all have a reverse phase backbone and are coupled with some kind of ion exchange moiety. Since chromatography in bioanalysis is usually performed in reverse phase, the ability to do sample preparation in the ion exchange dimension allows you to impart orthogonality into the overall bioanalytical method, giving you significant advantages in specificity, selectivity and reduction of matrix effects. For example, phospholipids will tend to bind to these mixed mode sorbents primarily by reverse phase, so if you are able to take advantage of that ion exchange moiety to bind your analyte, you get an elution that is very specific for those analytes. Furthermore, within each dimension of the sorbent, the reverse phase or mixed mode, you have the ability to narrow down the organic elution window to further minimize matrix effects eluting around your analyte – be it salts and other more polar constituents, or on the non-polar side, molecules like phospholipids. In addition, this dual retention nature allows you to remove, for example, acidic components from your basic analyte. The result is a very narrow selective window around your analyte and the minimization of matrix effects arising from many sources. This gives you an elution that is very specific and allows you to eliminate many sources of matrix effects.
A very strong coupling to these mixed-mode sorbents is the use of sub 2-micron LC. This is a strategy that we have employed in nearly every peptide bioanalytical method that we have developed. Sub 2-micron LC effectively allows you to fit more peaks in the same chromatographic space, minimizing the liklihood of co-elution, therefore reducing the potential for matrix effects that would suppress or enhance the analytes of interest. We published a paper in 2007 detailing how sub 2-micron particle LC has the ability to significantly reduce matrix effects [1]. Only in the past few years has the coupling of sub 2-micron LC and mixed mode sorbents come to the forefront and into use as a critical component of bioanalytical methods. I have to wonder if it correlates directly to the increase in the number of bioanalytical methods for large molecules. In my experience, these technologies are absolutely critical for successful development of bioanalytical methods for peptides and proteins, where you are challenged on so many levels to obtain accurate specificity and sensitivity.
You have recently published work with colleagues from King’s College London on analysis of insulin in human plasma using LC–MS [2]. What led you to develop an alternative to conventional approaches?
This is a very interesting piece of work for us and very exciting to work on. We looked at direct simultaneous quantification of intact human insulin and five of the most popular analogues in human plasma. It is unique in that we are analyzing insulins as intact species (about 6000 MW peptides), with no reduction or alkylation, and we are using analytical flow LC.
There are a couple of factors that led us down this path. First, if you look at the gold standard methodologies for quantifying insulin, these are typically ELISA or other ligand-binding assays. It is well documented that some of these assays for insulin generally suffer a lack of standardization and this is a big problem if you are trying to accurately and precisely quantify insulins in inter-laboratory studies or if you are trying to establish reference ranges. Also, many of the ELISAs or ligand-binding assays for insulin suffer cross-reactivity; they cannot all distinguish between human insulin and some of the analogues or metabolites. There is generally very poor linear dynamic range meaning a lot of dilution of samples has to occur in order to get them into the working range of the assay. With conventional LC–MS, you already have 4-5 orders of magnitude linear dynamic range from the technology and that easily accommodate a typical PK profile. In addition, we are looking at a method that could simultaneously measure, accurately quantify and differentiate six different insulins. Whereas with the gold standard ELISAs or ligand-binding assays, you can only analyze one insulin per assay, so it is very labor intensive.
Finally, a lot of laboratories are already equipped with LCMS and have personnel who are comfortable with it. Many of the most successful drugs on the market are insulin analogues and several are shortly coming off patent, so we are seeing a big surge in the requirement for insulin analysis, either as replacement insulins are developed or the initial drug developers are trying to extend patent life. So there is a really high demand from the industry for an LC–MS-based assay.
How did you achieve the required sensitivity and selectivity?
The insulin assay is one where every piece of the methodology (from sample pretreatment to the sample preparation, the chromatography and the MS) all had to be very carefully optimized and researched. No single piece could stand alone to make the methodology work. If we start from the MS side of it, the key here was to use higher m/z precursors where possible. For example, if I am looking at the 6th charge state of an insulin versus the 7th charge state, with the 7th being at lower m/z, I would get more specificity out of the 6th charge state, so using higher m/z but a lower charge state. Typically if I am working at an m/z above about 1000 or so then I tend to get significantly better specificity than working in the m/z range of 800 or below. This is something that we have only been able to do successfully in recent years with some of the current instrumentation that has excellent sensitivity above m/z of 1000. In this particular study we used the Waters TQS, which has a mass range of up to 2048. In the publication you will see a few examples demonstrating the night and day difference between using some of these higher m/z compared with using some of the m/z around 800 range. We are really fortunate to be able to take advantage of that sensitivity and the specificity through the use of these higher m/z precursors and fragment pairs.
The next piece of the puzzle is the sample preparation component. Here we optimized an initial protein precipitation pretreatment step where we tuned in the ratio of organic to plasma, in order to precipitate the high abundance proteins (e.g. serum albumins, immunoglobulins) while keeping the insulins in solution. This eliminated a lot of the proteins that would potentially have MS envelopes that could overlap or interfere with the precursors for the analytes. We followed this pretreatment by mixed mode SPE, which as mentioned earlier, is one of the most selective ways of doing an extraction for bioanalysis. So we now have the orthogonality of mixed mode SPE incorporated into the method.
The last piece that was really critical was the chromatography segment. We implemented a very recent column choice, a solid core positively charged surface column (CORTECS) that is superficially porous. This column maximizes the mass transfer of a large compound like insulin, the superficially porous nature means it doesn’t migrate into the pores like it would with a fully porous particle. The result is a very high efficiency separation, and from a practical standpoint what that means for the end user is very narrow peaks and improved signal-to-noise versus other column technologies. For an application such as insulin (where you are really pushing the limits of sensitivity) it is critical to be able to get everything you can out of the signal-to-noise and the sensitivity, and we were able to do that with this particular column. The final step is the inclusion of a trap and back elute strategy, whereby we focus the diluted insulin band on the head of a trap column, flush away some of the interferences and then back elute onto the analytical column.
All of these stages listed together may sound complex, but the final product is 96-well SPE (something that can be automated), analytical LC and a Xevo TQS (a triple quadrupole mass spectrometer used by many laboratories). Therefore, you have a technology that is fully accessible versus some of the historical methods that not only would have taken a lot more time and induce potential variability, but also require the appropriate expertise.
What are you excited about working on over the next year?
We have some exciting things coming up in our Alzheimer’s research as we continue to work with the Global Biomarkers Standardization Committee and the Alzheimer’s Association to evaluate methods for the amyloid beta 1-42 isoform as a putative biomarker. We expect some future continued applications of the insulin methodology. We are also looking at some work associated with alternative scales of chromatography that really has the potential to revolutionize the bioanalytical world in terms of what these alternative scale chromatographic integrated systems are able to do.
References
1. Chambers E, Wagrowski-Diehl DM, Lu Z, Mazzeo JR. Systematic and comprehensive strategy for reducing matrix effects in LC/MS/MS analyses. J. Chromatogr. B 852(1–2), 22–34 (2007).
2. Chambers E, Fountain K, Smith N et al. Multidimensional LC/MS/MS Enables Simultaneous Quantification of Intact Human Insulin and 5 Recombinant Analogs in Human Plasma. Anal.Chem. 86(1), 694–702 (2014).